Thank you for visiting nature.com. You are using a browser version with limited support for CSS. To obtain the best experience, we recommend you use a more up to date browser (or turn off compatibility mode in Internet Explorer). In the meantime, to ensure continued support, we are displaying the site without styles and JavaScript.
- View all journals
- Explore content
- About the journal
- Publish with us
- Sign up for alerts
- Review Article
- Published: 31 January 2023

Global water resources and the role of groundwater in a resilient water future
- Bridget R. Scanlon ORCID: orcid.org/0000-0002-1234-4199 1 ,
- Sarah Fakhreddine 1 , 2 ,
- Ashraf Rateb 1 ,
- Inge de Graaf ORCID: orcid.org/0000-0001-7748-868X 3 ,
- Jay Famiglietti 4 ,
- Tom Gleeson 5 ,
- R. Quentin Grafton 6 ,
- Esteban Jobbagy 7 ,
- Seifu Kebede 8 ,
- Seshagiri Rao Kolusu 9 ,
- Leonard F. Konikow 10 ,
- Di Long ORCID: orcid.org/0000-0001-9033-5039 11 ,
- Mesfin Mekonnen ORCID: orcid.org/0000-0002-3573-9759 12 ,
- Hannes Müller Schmied 13 , 14 ,
- Abhijit Mukherjee 15 ,
- Alan MacDonald ORCID: orcid.org/0000-0001-6636-1499 16 ,
- Robert C. Reedy 1 ,
- Mohammad Shamsudduha 17 ,
- Craig T. Simmons 18 ,
- Alex Sun 1 ,
- Richard G. Taylor 19 ,
- Karen G. Villholth 20 ,
- Charles J. Vörösmarty 21 &
- Chunmiao Zheng ORCID: orcid.org/0000-0001-5839-1305 22
Nature Reviews Earth & Environment volume 4 , pages 87–101 ( 2023 ) Cite this article
15k Accesses
152 Citations
289 Altmetric
Metrics details
- Hydrogeology
- Water resources
An Author Correction to this article was published on 29 March 2023
This article has been updated
Water is a critical resource, but ensuring its availability faces challenges from climate extremes and human intervention. In this Review, we evaluate the current and historical evolution of water resources, considering surface water and groundwater as a single, interconnected resource. Total water storage trends have varied across regions over the past century. Satellite data from the Gravity Recovery and Climate Experiment (GRACE) show declining, stable and rising trends in total water storage over the past two decades in various regions globally. Groundwater monitoring provides longer-term context over the past century, showing rising water storage in northwest India, central Pakistan and the northwest United States, and declining water storage in the US High Plains and Central Valley. Climate variability causes some changes in water storage, but human intervention, particularly irrigation, is a major driver. Water-resource resilience can be increased by diversifying management strategies. These approaches include green solutions, such as forest and wetland preservation, and grey solutions, such as increasing supplies (desalination, wastewater reuse), enhancing storage in surface reservoirs and depleted aquifers, and transporting water. A diverse portfolio of these solutions, in tandem with managing groundwater and surface water as a single resource, can address human and ecosystem needs while building a resilient water system.
Net trends in total water storage data from the GRACE satellite mission range from −310 km 3 to 260 km 3 total over a 19-year record in different regions globally, caused by climate and human intervention.
Groundwater and surface water are strongly linked, with 85% of groundwater withdrawals sourced from surface water capture and reduced evapotranspiration, and the remaining 15% derived from aquifer depletion.
Climate and human interventions caused loss of ~90,000 km 2 of surface water area between 1984 and 2015, while 184,000 km 2 of new surface water area developed elsewhere, primarily through filling reservoirs.
Human intervention affects water resources directly through water use, particularly irrigation, and indirectly through land-use change, such as agricultural expansion and urbanization.
Strategies for increasing water-resource resilience include preserving and restoring forests and wetlands, and conjunctive surface water and groundwater management.
This is a preview of subscription content, access via your institution
Access options
Access Nature and 54 other Nature Portfolio journals
Get Nature+, our best-value online-access subscription
24,99 € / 30 days
cancel any time
Subscribe to this journal
Receive 12 digital issues and online access to articles
92,52 € per year
only 7,71 € per issue
Buy this article
- Purchase on Springer Link
- Instant access to full article PDF
Prices may be subject to local taxes which are calculated during checkout
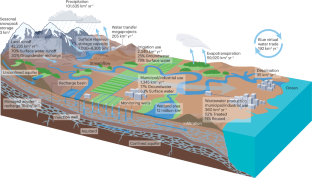
Similar content being viewed by others
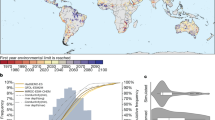
Environmental flow limits to global groundwater pumping
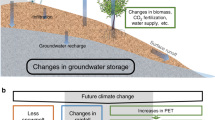
Divergent effects of climate change on future groundwater availability in key mid-latitude aquifers
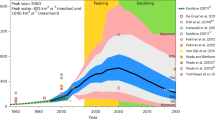
Global peak water limit of future groundwater withdrawals
Change history, 29 march 2023.
A Correction to this paper has been published: https://doi.org/10.1038/s43017-023-00418-9
Vorosmarty, C. J. et al. Global threats to human water security and river biodiversity. Nature 467 , 555–561 (2010).
Article Google Scholar
Doell, P., Mueller Schmied, H., Schuh, C., Portmann, F. T. & Eicker, A. Global-scale assessment of groundwater depletion and related groundwater abstractions: combining hydrological modeling with information from well observations and GRACE satellites. Water Resour. Res. 50 , 5698–5720 (2014).
Wada, Y. et al. Global depletion of groundwater resources. Geophys. Res. Lett. 37 , L20402 (2010).
Douville, H. et al. in Climate Change 2021: The Physical Science Basis (eds Masson-Delmotte, V. et al.) 1055–1210 (IPCC, Cambridge Univ. Press, 2021).
Olivier, D. W. & Xu, Y. X. Making effective use of groundwater to avoid another water supply crisis in Cape Town, South Africa. Hydrogeol. J. 27 , 823–826 (2019).
Ozment, S. et al. Natural infrastructure in Sao Paulo’s water system. World Resources Institute Report 2013–2014: Interim Findings (2018).
Pascale, S., Kapnick, S. B., Delworth, T. L. & Cooke, W. F. Increasing risk of another Cape Town ‘Day Zero’ drought in the 21st century. Proc. Natl Acad. Sci. USA 117 , 29495 (2020).
Alley, W. M., Reilly, T. E. & Franke, O. L. Sustainability of ground-water resources. US Geological Survey Circular 1186 (1999).
Breslin, S. COP26 has 4 goals. Water is central to all of them. SIWI News https://siwi.org/latest/cop26-has-4-goals-water-is-central-to-all-of-them/ (2021).
Global Risks 2021 16th edition (World Economic Forum, 2021); https://www.weforum.org/reports/the-global-risks-report-2021/
The Water Challenge: The Roundtable on Water Financing (OECD, 2022); https://www.oecd.org/water/roundtable-on-financing-water.htm
The United Nations World Water Development Report 2018: Nature-Based Solutions for Water (United Nations World Water Assessment Program/UNESCO, 2018).
Browder, G., Ozment, S., Rehberger-Bescos, I., Gartner, T. & Lange, G. M. Integrating Green and Gray: Creating Next Generation Infrastructure (World Bank and World Resources Institute, 2019); https://openknowledge.worldbank.org/handle/10986/31430
Making Every Drop Count: Agenda for Water Action (High Level Panel on Water, United Nations and World Bank, 2018).
Lederer, E. M. Next UN assembly president warns world in dangerous crisis. Washington Post https://www.washingtonpost.com/world/next-un-assembly-president-warns-world-in-dangerous-crisis/2022/06/07/55075dce-e6b6-11ec-a422-11bbb91db30b_story.html (7 June 2022).
Tapley, B. D. et al. Contributions of GRACE to understanding climate change. Nat. Clim. Change 9 , 358–369 (2019).
Wada, Y. & Bierkens, M. F. P. Sustainability of global water use: past reconstruction and future projections. Environ. Res. Lett. https://doi.org/10.1088/1748-9326/9/10/104003 (2014).
Mekonnen, M. M. & Hoekstra, A. Y. Blue water footprint linked to national consumption and international trade is unsustainable. Nat. Food 1 , 792–800 (2020).
Rodell, M. et al. Emerging trends in global freshwater availability. Nature 557 , 651–659 (2018).
Save, H., Bettadpur, S. & Tapley, B. D. High-resolution CSR GRACE RL05 mascons. J. Geophys. Res. Solid Earth 121 , 7547–7569 (2016).
Tapley, B. D., Bettadpur, S., Watkins, M. & Reigber, C. The Gravity Recovery And Climate Experiment: mission overview and early results. Geophys. Res. Lett. https://doi.org/10.1029/2004gl019920 (2004).
Richey, A. S. et al. Quantifying renewable groundwater stress with GRACE. Water Resour. Res. 51 , 5217–5238 (2015).
Shamsudduha, M. & Taylor, R. G. Groundwater storage dynamics in the world’s large aquifer systems from GRACE: uncertainty and role of extreme precipitation. Earth Syst. Dyn. 11 , 755–774 (2020).
Vishwakarma, B. D., Bates, P., Sneeuw, N., Westaway, R. M. & Bamber, J. L. Re-assessing global water storage trends from GRACE time series. Environ. Res. Lett. 16 , 034005 (2021).
Pekel, J. F., Cottam, A., Gorelick, N. & Belward, A. S. High-resolution mapping of global surface water and its long-term changes. Nature 540 , 418–436 (2016).
Lehner, B. et al. High-resolution mapping of the world’s reservoirs and dams for sustainable river-flow management. Front. Ecol. Environ. 9 , 494–502 (2011).
Scanlon, B. R. et al. Global models underestimate large decadal declining and rising water storage trends relative to GRACE satellite data. Proc. Natl Acad. Sci. USA 115 , E1080–E1089 (2018).
Winter, T. C., Harvey, J. W., Franke, O. L. & Alley, W. M. Ground Water and Surface Water: A Single Resource . Circular 1139 (United States Geological Survey, 1998).
Konikow, L. F. Overestimated water storage. Nat. Geosci. 6 , 3 (2013).
Konikow, L. F. Contribution of global groundwater depletion since 1900 to sea-level rise. Geophys. Res. Lett. https://doi.org/10.1029/2011gl048604 (2011).
Pokhrel, Y. N. et al. Model estimates of sea-level change due to anthropogenic impacts on terrestrial water storage. Nat. Geosci. 5 , 389–392 (2012).
de Graaf, I. E. M. et al. A global-scale two-layer transient groundwater model: development and application to groundwater depletion. Adv. Water Resour. 102 , 53–67 (2017).
Rateb, A. et al. Comparison of groundwater storage changes from GRACE satellites with monitoring and modeling of major U.S. aquifers. Water Resour. Res. https://doi.org/10.1029/2020WR027556 (2020).
de Graaf, I. E. M., Gleeson, T., van Beek, L. P. H., Sutanudjaja, E. H. & Bierkens, M. F. P. Environmental flow limits to global groundwater pumping. Nature 574 , 90–94 (2019).
Sophocleous, M. From safe yield to sustainable development of water resources — the Kansas experience. J. Hydrol. 235 , 27–43 (2000).
Konikow, L. F. & Bredehoeft, J. D. Groundwater Resource Development: Effects and Sustainability (The Groundwater Project, 2020).
MacAllister, D. J., Krishan, G., Basharat, M., Cuba, D. & MacDonald, A. M. A century of groundwater accumulation in Pakistan and northwest India. Nat. Geosci. https://doi.org/10.1038/s41561-022-00926-1 (2022).
Scanlon, B. R. et al. Effects of climate and irrigation on GRACE-based estimates of water storage changes in major US aquifers. Environ. Res. Lett. https://doi.org/10.1088/1748-9326/ac16ff (2021).
McGuire, V. L. Water-Level and Recoverable Water In Storage Changes, High Plains Aquifer, Predevelopment to 2015 and 2013–15 . US Geological Survey Scientific Investigations Report 2017–5040 (2017); https://doi.org/10.3133/sir20175040
Faunt, C. C. Groundwater availability of the Central Valley Aquifer, California. US Geol. Surv. Prof. Pap . 1766 (2009).
Vorosmarty, C. J., Green, P., Salisbury, J. & Lammers, R. B. Global water resources: vulnerability from climate change and population growth. Science 289 , 284–288 (2000).
Mekonnen, M. M. & Hoekstra, A. Y. Four billion people facing severe water scarcity. Sci. Adv. https://doi.org/10.1126/sciadv.1500323 (2016).
Vorosmarty, C. J. & Sahagian, D. Anthropogenic disturbance of the terrestrial water cycle. Bioscience 50 , 753–765 (2000).
Gronwall, J. & Danert, K. Regarding groundwater and drinking water access through a human rights lens: self-supply as a norm. Water https://doi.org/10.3390/w12020419 (2020).
van Vliet, M. T. H. et al. Global water scarcity including surface water quality and expansions of clean water technologies. Environ. Res. Lett. https://doi.org/10.1088/1748-9326/abbfc3 (2021).
Podgorski, J. & Berg, M. Global threat of arsenic in groundwater. Science 368 , 845–850 (2020).
Yapiyev, V., Sagintayev, Z., Inglezakis, V. J., Samarkhanov, K. & Verhoef, A. Essentials of endorheic basins and lakes: a review in the context of current and future water resource management and mitigation activities in Central Asia. Water https://doi.org/10.3390/w9100798 (2017).
Pauloo, R. A., Fogg, G. E., Guo, Z. L. & Harter, T. Anthropogenic basin closure and groundwater salinization (ABCSAL). J. Hydrol. https://doi.org/10.1016/j.jhydrol.2020.125787 (2021).
Cao, T. Z., Han, D. M. & Song, X. F. Past, present, and future of global seawater intrusion research: a bibliometric analysis. J. Hydrol. https://doi.org/10.1016/j.jhydrol.2021.126844 (2021).
Werner, A. D. et al. Seawater intrusion processes, investigation and management: recent advances and future challenges. Adv. Water Resour. 51 , 3–26 (2013).
Held, I. M. & Soden, B. J. Robust responses of the hydrological cycle to global warming. J. Clim. 19 , 5686–5699 (2006).
Fan, X., Duan, Q. Y., Shen, C. P., Wu, Y. & Xing, C. Global surface air temperatures in CMIP6: historical performance and future changes. Environ. Res. Lett. 15 , 104056 (2020).
Tabari, H. Climate change impact on flood and extreme precipitation increases with water availability. Sci. Rep. https://doi.org/10.1038/s41598-020-70816-2 (2020).
Williams, A. P. et al. Large contribution from anthropogenic warming to an emerging North American megadrought. Science 368 , 314 (2020).
Arias, P. A. et al. in Climate Change 2021: The Physical Science Basis (eds Masson-Delmotte, V. et al.) 33−144 (IPCC, Cambridge Univ. Press, 2021).
van Dijk, A. et al. The Millennium Drought in southeast Australia (2001–2009): natural and human causes and implications for water resources, ecosystems, economy, and society. Water Resour. Res. 49 , 1040–1057 (2013).
Scanlon, B. R. et al. Hydrologic implications of GRACE satellite data in the Colorado River Basin. Water Resour. Res. 51 , 9891–9903 (2015).
Rateb, A., Scanlon, B. R. & Kuo, C. Y. Multi-decadal assessment of water budget and hydrological extremes in the Tigris-Euphrates Basin using satellites, modeling, and in-situ data. Sci. Total Environ. https://doi.org/10.1016/j.scitotenv.2020.144337 (2021).
Anyamba, A., Glennie, E. & Small, J. Teleconnections and interannual transitions as observed in African vegetation: 2015–2017. Remote Sens. https://doi.org/10.3390/rs10071038 (2018).
Scanlon, B. R. et al. Linkages between GRACE water storage, hydrologic extremes, and climate teleconnections in major African aquifers. Environ. Res. Lett. https://doi.org/10.1088/1748-9326/ac3bfc (2022).
Ul Hassan, W. & Nayak, M. A. Global teleconnections in droughts caused by oceanic and atmospheric circulation patterns. Environ. Res. Lett. https://doi.org/10.1088/1748-9326/abc9e2 (2021).
Shen, Z. X. et al. Drying in the low-latitude Atlantic Ocean contributed to terrestrial water storage depletion across Eurasia. Nat. Commun. 13 , 1849 (2022).
Dettinger, M. D. Atmospheric rivers as drought busters on the US West Coast. J. Hydrometeorol. 14 , 1721–1732 (2013).
Taylor, R. G. et al. Ground water and climate change. Nat. Clim. Change 3 , 322–329 (2013).
Cuthbert, M. O. et al. Observed controls on resilience of groundwater to climate variability in sub-Saharan Africa. Nature 572 , 230 (2019).
Hugonnet, R. et al. Accelerated global glacier mass loss in the early twenty-first century. Nature 592 , 726 (2021).
Zhao, F., Long, D., Li, X., Huang, Q. & Han, P. Rapid glacier mass loss in the Southeastern Tibetan Plateau since the year 2000 from satellite observations. Remote. Sens. Environ. 270 , 112853 (2022).
Li, X. Y. et al. Climate change threatens terrestrial water storage over the Tibetan Plateau. Nat. Clim. Change https://doi.org/10.1038/s41558-022-01443-0 (2022).
Yao, T. D. et al. The imbalance of the Asian water tower. Nat. Rev. Earth Environ. https://doi.org/10.1038/s43017-022-00299-4 (2022).
Immerzeel, W. W., van Beek, L. P. H. & Bierkens, M. F. P. Climate change will affect the Asian water towers. Science 328 , 1382–1385 (2010).
Immerzeel, W. W. et al. Importance and vulnerability of the world’s water towers. Nature 577 , 364 (2020).
Dery, S. J. et al. Detection of runoff timing changes in pluvial, nival, and glacial rivers of western Canada. Water Resour. Res. https://doi.org/10.1029/2008wr006975 (2009).
Siebert, S. et al. Groundwater use for irrigation – a global inventory. Hydrol. Earth Syst. Sci. 7 , 3977–4021 (2010).
Google Scholar
Scanlon, B. R. et al. Groundwater depletion and sustainability of irrigation in the US High Plains and Central Valley. Proc. Natl Acad. Sci. USA 109 , 9320–9325 (2012).
Dahlke, H. E. et al. in Advanced Tools for Integrated Water Resources Management Vol. 3 (eds Friesen, J. & Rodriguez-Sinobas, L.) 215–275 (Elsevier, 2018).
Reddy, V. R., Pavelic, P. & Hanjra, M. A. Underground taming of floods for irrigation (UTFI) in the river basins of South Asia: institutionalising approaches and policies for sustainable water management and livelihood enhancement. Water Policy 20 , 369–387 (2018).
McDonald, R. I., Weber, K. F., Padowski, J., Boucher, T. & Shemie, D. Estimating watershed degradation over the last century and its impact on water-treatment costs for the world’s large cities. Proc. Natl Acad. Sci. USA 113 , 9117–9122 (2016).
The State of the World’s Forests 2020. Forests, Biodiversity, and Peopl e (FAO/UNEP, 2020).
Convention on Wetlands. Global Wetland Outlook: Special Edition 2021 (Secretariat of the Convention on Wetlands, 2021).
Scanlon, B. R., Jolly, I., Sophocleous, M. & Zhang, L. Global impacts of conversions from natural to agricultural ecosystems on water resources: quantity versus quality. Water Resour. Res. https://doi.org/10.1029/2006WR005486 (2007).
Nosetto, M. D., Paez, R. A., Ballesteros, S. I. & Jobbagy, E. G. Higher water-table levels and flooding risk under grain vs. livestock production systems in the subhumid plains of the Pampas. Agric. Ecosyst. Environ. 206 , 60–70 (2015).
Favreau, G. et al. Land clearing, climate variability, and water resources increase in semiarid southwest Niger: a review. Water Resour. Res. https://doi.org/10.1029/2007wr006785 (2009).
Walker, C. D., Zhang, l, Ellis, T. W., Hatton, T. J. & Petheram, C. Estimating impacts of changed land use on recharge: review of modelling and other approaches appropriate for management of dryland salinity. Hydrogeol. J. 10 , 68–90 (2002).
Nosetto, M. D., Jobbagy, E. G., Jackson, R. B. & Sznaider, G. A. Reciprocal influence of crops and shallow ground water in sandy landscapes of the Inland Pampas. Field Crops Res. 113 , 138–148 (2009).
Gimenez, R., Mercau, J., Nosetto, M., Paez, R. & Jobbagy, E. The ecohydrological imprint of deforestation in the semiarid Chaco: insights from the last forest remnants of a highly cultivated landscape. Hydrol. Process. 30 , 2603–2616 (2016).
Eilers, R. G., Eilers, W. D. & Fitzgerald, M. M. A salinity risk index for soils of the Canadian prairies. Hydrogeol. J. 5 , 68–79 (1997).
Progress on Household Drinking Water, Sanitation and Hygiene 2000–2020: Five Years into the SDGs (WHO/UNICEF, 2021).
Cobbing, J. & Hiller, B. Waking a sleeping giant: realizing the potential of groundwater in sub-Saharan Africa. World Dev. 122 , 597–613 (2019).
Rockström, J. & Falkenmark, M. Agriculture: increase water harvesting in Africa. Nature 519 , 283–285 (2015).
MacAllister, D. J., MacDonald, A. M., Kebede, S., Godfrey, S. & Calow, R. Comparative performance of rural water supplies during drought. Nat. Commun. 11 , 1099 (2020).
Aboah, M. & Miyittah, M. K. Estimating global water, sanitation, and hygiene levels and related risks on human health, using global indicators data from 1990 to 2020. J. Water Health 20 , 1091–1101 (2022).
Abell, R. et al. Beyond the Source: The Environmental, Economic and Community Benefits of Source Water Protection (The Nature Conservancy, 2017).
Herrera-Garcia, G. et al. Mapping the global threat of land subsidence. Science 371 , 34–36 (2021).
Scanlon, B. R., Reedy, R. C., Faunt, C. C., Pool, D. & Uhlman, K. Enhancing drought resilience with conjunctive use and managed aquifer recharge in California and Arizona. Environ. Res. Lett. 11 , 035013 (2016).
Qadir, M. et al. Global and regional potential of wastewater as a water, nutrient and energy source. Nat. Resour. Forum 44 , 40–51 (2020).
Water Reuse within a Circular Economy Context . Global Water Security Issues Series 2 (UNESCO, 2020).
Jones, E. R., van Vliet, M. T. H., Qadir, M. & Bierkens, M. F. P. Country-level and gridded estimates of wastewater production, collection, treatment and reuse. Earth Syst. Sci. Data 13 , 237–254 (2021).
Jeuland, M. Challenges to wastewater reuse in the Middle East and North Africa. Middle East. Dev. J. 7 , 1–25 (2015).
Zhang, Y. & Shen, Y. Wastewater irrigation: past, present, and future. WIREs Water 6 , e1234 (2019).
Fito, J. & Van Hulle, S. W. H. Wastewater reclamation and reuse potentials in agriculture: towards environmental sustainability. Environ. Dev. Sust. 23 , 2949–2972 (2021).
Gao, L., Yoshikawa, S., Iseri, Y., Fujimori, S. & Kanae, S. An economic assessment of the global potential for seawater desalination to 2050. Water https://doi.org/10.3390/w9100763 (2017).
Ahdab, Y. D., Thiel, G. P., Bohlke, J. K., Stanton, J. & Lienhard, J. H. Minimum energy requirements for desalination of brackish groundwater in the United States with comparison to international datasets. Water Res. 141 , 387–404 (2018).
Jones, E., Qadir, M., van Vliet, M. T. H., Smakhtin, V. & Kang, S. M. The state of desalination and brine production: a global outlook. Sci. Total Environ. 657 , 1343–1356 (2019).
Lin, S. S. et al. Seawater desalination technology and engineering in China: a review. Desalination https://doi.org/10.1016/j.desal.2020.114728 (2021).
Martinez-Alvarez, V., Martin-Gorriz, B. & Soto-Garcia, M. Seawater desalination for crop irrigation — a review of current experiences and revealed key issues. Desalination 381 , 58–70 (2016).
Smith, K., Liu, S. M., Hu, H. Y., Dong, X. & Wen, X. H. Water and energy recovery: the future of wastewater in China. Sci. Total Environ. 637 , 1466–1470 (2018).
Pulido-Bosch, A. et al. Impacts of agricultural irrigation on groundwater salinity. Environ/ Earth Sci. https://doi.org/10.1007/s12665-018-7386-6 (2018).
Kurnik, J. The Next California: Phase 1: Investigating Potential in the Mid-Mississippi Delta River Region (The Markets Institute at WWF, 2020); https://www.worldwildlife.org/publications/the-next-california-phase-1-investigating-potential-in-the-mid-mississippi-delta-river-region
Senay, G. B., Schauer, M., Friedrichs, M., Velpuri, N. M. & Singh, R. K. Satellite-based water use dynamics using historical Landsat data (1984–2014) in the southwestern United States. Remote Sens. Environ. 202 , 98–112 (2017).
Gebremichael, M., Krishnamurthy, P. K., Ghebremichael, L. T. & Alam, S. What drives crop land use change during multi-year droughts in California’s Central Valley? Prices or concern for water? Remote Sens. https://doi.org/10.3390/rs13040650 (2021).
Brauman, K. A., Siebert, S. & Foley, J. A. Improvements in crop water productivity increase water sustainability and food security — a global analysis. Environ. Res. Lett. 8 , 024030 (2013).
Mekonnen, M. M., Hoekstra, A. Y., Neale, C. M. U., Ray, C. & Yang, H. S. Water productivity benchmarks: the case of maize and soybean in Nebraska. Agric. Water Manag. https://doi.org/10.1016/j.agwat.2020.106122 (2020).
Colaizzi, P. D., Gowda, P. H., Marek, T. H. & Porter, D. O. Irrigation in the Texas High Plains: a brief history and potential reductions in demand. Irrig. Drain. 58 , 257–274 (2008).
Scanlon, B. R., Gates, J. B., Reedy, R. C., Jackson, A. & Bordovsky, J. Effects of irrigated agroecosystems: (2). Quality of soil water and groundwater in the southern High Plains, Texas. Water Resour. Res. 46 , W09538 (2010).
Ward, F. A. & Pulido-Velazquez, M. Water conservation in irrigation can increase water use. Proc. Natl Acad. Sci. USA 105 , 18215–18220 (2008).
Grafton, R. Q. et al. The paradox of irrigation efficiency. Science 361 , 748–750 (2018).
Alcott, B. in The Jevons Paradox and the Myth of Resource Efficiency Improvements (eds Polimeni, J. M., Mayumi, K., & Giampetro, M.) 7–78 (Earthscan, 2008).
Aarnoudse, E. & Bluemling, B. Controlling Groundwater Through Smart Card Machines: The Case of Water Quotas and Pricing Mechanisms in Gansu Province, China . Groundwater Solutions Initiative for Policy and Practice (GRIPP) Case Profile Series 02 (International Water Management Institute, 2017); https://doi.org/10.5337/2016.224
Kinzelbach, W., Wang, H., Li, Y., Wang, L. & Li, N. Groundwater Overexploitation in the North China Plain: A Path to Sustainability (Springer, 2021).
McDougall, R., Kristiansen, P. & Rader, R. Small-scale urban agriculture results in high yields but requires judicious management of inputs to achieve sustainability. Proc. Natl Acad. Sci. USA 116 , 129–134 (2019).
Langemeyer, J., Madrid-Lopez, C., Mendoza Beltran, A. & Villalba Mendez, G. Urban agriculture — a necessary pathway towards urban resilience and global sustainability? Landsc. Urban Plan. 210 , 104055 (2021).
Palmer, L. Urban agriculture growth in US cities. Nat. Sust. 1 , 5–7 (2018).
Grafius, D. R. et al. Estimating food production in an urban landscape. Sci. Rep. 10 , 5141 (2020).
The State of Food Insecurity in the World 2015 (FAO/IFAD/WFP, 2015).
Kummu, M. et al. Lost food, wasted resources: global food supply chain losses and their impacts on freshwater, cropland, and fertiliser use. Sci. Total Environ. 438 , 477–489 (2012).
Gleick, P. H. Global freshwater resources: soft-path solutions for the 21st century. Science 302 , 1524–1528 (2003).
Miralles-Wilhelm, F. Nature-Based Solutions in Agriculture — Sustainable Management and Conservation of Land, Water, and Biodiversity (FAO/The Nature Conservancy, 2021).
McDonald, R. I. & Shemie, D. Urban Water Blueprint: Mapping Conservation Solutions to the Global Water Challenge (The Nature Conservancy, 2014); http://water.nature.org/waterblueprint
Kane, M. & Erickson, J. D. Urban metabolism and payment for ecosystem services: history and policy analysis of the New York city water supply. Adv. Econ. Environ. Resour. 7 , 307–328 (2007).
Greater Cape Town Water Fund: Business Case: Assessing the Return on Investment for Ecological Infrastructure Restoration (The Nature Conservancy, 2019).
Hu, J., Lu, Y. H., Fu, B. J., Comber, A. J. & Harris, P. Quantifying the effect of ecological restoration on runoff and sediment yields: a meta-analysis for the Loess Plateau of China. Prog. Phys. Geogr. Earth Environ. 41 , 753–774 (2017).
Liu, W. W. et al. Improving wetland ecosystem health in China. Ecol. Indic. https://doi.org/10.1016/j.ecolind.2020.106184 (2020).
Cities100: Chennai Is Restoring Waterbodies to Protect Against Flooding and Drought . C40 Knowledge Hub: Nordic Sustainability, South and West Asia, Chennai, Case Studies and Best Practice Examples https://www.c40knowledgehub.org/s/article/Cities100-Chennai-is-restoring-waterbodies-to-protect-against-flooding-and-drought?language=en_US (2019).
Chung, M. G., Frank, K. A., Pokhrel, Y., Dietz, T. & Liu, J. G. Natural infrastructure in sustaining global urban freshwater ecosystem services. Nat. Sust. 4 , 1068 (2021).
Qi, Y. F. et al. Addressing challenges of urban water management in Chinese sponge cities via nature-based solutions. Water https://doi.org/10.3390/w12102788 (2020).
Acreman, M. et al. Evidence for the effectiveness of nature-based solutions to water issues in Africa. Environ. Res. Lett. https://doi.org/10.1088/1748-9326/ac0210 (2021).
Livneh, B. & Badger, A. M. Drought less predictable under declining future snowpack. Nat. Clim. Change 10 , 452–458 (2020).
Mulligan, M., van Soesbergen, A. & Sáenz, L. GOODD, a global dataset of more than 38,000 georeferenced dams. Sci. Data 7 , 31 (2020).
International Commission on Large Dams https://www.icold-cigb.org/ (2022).
Yang, G., Guo, S., Liu, P. & Block, P. Integration and evaluation of forecast-informed multiobjective reservoir operations. J. Water Resour. Plan. Manag. 146 , 04020038 (2020).
Delaney, C. J. et al. Forecast informed reservoir operations using ensemble streamflow predictions for a multipurpose reservoir in northern California. Water Resour. Res . https://doi.org/10.1029/2019wr026604 (2020).
Amarasinghe, U. A., Muthuwatta, L., Surinaidu, L., Anand, S. & Jain, S. K. Reviving the Ganges water machine: potential. Hydrol. Earth Syst. Sci. 20 , 1085–1101 (2016).
Shamsudduha, M. et al. The Bengal water machine: quantified freshwater capture in Bangladesh. Science 377 , 1315–1319 (2022).
Chao, B. F., Wu, Y. H. & Li, Y. S. Impact of artificial reservoir water impoundment on global sea level. Science 320 , 212–214 (2008).
Zarfl, C., Lumsdon, A. E., Berlekamp, J., Tydecks, L. & Tockner, K. A global boom in hydropower dam construction. Aquat. Sci. 77 , 161–170 (2015).
Zarfl, C. et al. Future large hydropower dams impact global freshwater megafauna. Sci. Rep. https://doi.org/10.1038/s41598-019-54980-8 (2019).
Wheeler, K. G., Jeuland, M., Hall, J. W., Zagona, E. & Whittington, D. Understanding and managing new risks on the Nile with the Grand Ethiopian Renaissance Dam. Nat. Commun. https://doi.org/10.1038/s41467-020-19089-x (2020).
Di Baldassarre, G. et al. Water shortages worsened by reservoir effects. Nat. Sust. 1 , 617–622 (2018).
Dahlke, H. E., Brown, A. G., Orloff, S., Putnam, D. & O’Geen, T. Managed winter flooding of alfalfa recharges groundwater with minimal crop damage. Calif. Agric. 72 , 65–75 (2018).
Yang, Q. & Scanlon, B. R. How much water can be captured from flood flows to store in depleted aquifers for mitigating floods and droughts? A case study from Texas, US. Environ. Res. Lett. 14 , 054011 (2019).
Dillon, P. et al. Sixty years of global progress in managed aquifer recharge. Hydrogeol. J. https://doi.org/10.1007/s10040-018-1841-z. (2018).
Groundwater Replenishment System Technical Brochure, https://www.ocwd.com/media/10443/gwrs-technical-brochure-2021.pdf (2021).
Konikow, L. F. Groundwater Depletion in the United States (1900–2008) . US Geological Survey Scientific Investigation Report 2013–5079, http://pubs.usgs.gov/sir/2013/5079 (2013).
Hartog, N. & Stuyfzand, P. J. Water quality donsiderations on the rise as the use of managed aquifer recharge systems widens. Water 9 , 808 (2017).
Shumilova, O., Tockner, K., Thieme, M., Koska, A. & Zarfl, C. Global water transfer megaprojects: a potential solution for the water–food–energy nexus? Front. Environ. Sci. https://doi.org/10.3389/fenvs.2018.00150 (2018).
Long, D. et al. South-to-north water diversion stabilizing Beijing’s groundwater levels. Nat. Commun. https://doi.org/10.1038/s41467-020-17428-6 (2020).
Zhuang, W. Eco-environmental impact of inter-basin water transfer projects: a review. Environ. Sci. Pollut. Res. 23 , 12867–12879 (2016).
Hoekstra, A. Y. Virtual Water Trade : Proceedings of the International Expert Meeting on Virtual Water Trade (UNESCO-IHE, 2003).
Oki, T. & Kanae, S. Virtual water trade and world water resources. Water Sci. Technol. 49 , 203–209 (2004).
Dolan, F. et al. Evaluating the economic impact of water scarcity in a changing world. Nat. Commun. https://doi.org/10.1038/s41467-021-22194-0 (2021).
Hoekstra, A. Y. & Mekonnen, M. M. The water footprint of humanity. Proc. Natl Acad. Sci. USA 109 , 3232–3237 (2012).
Dalin, C., Wada, Y., Kastner, T. & Puma, M. J. Groundwater depletion embedded in international food trade. Nature 543 , 700–704 (2017).
Hanasaki, N., Inuzuka, T., Kanae, S. & Oki, T. An estimation of global virtual water flow and sources of water withdrawal for major crops and livestock products using a global hydrological model. J. Hydrol. 384 , 232–244 (2010).
Mekonnen, M. M. & Gerbens-Leenes, W. The water footprint of global food production. Water https://doi.org/10.3390/w12102696 (2020).
Australian Water Markets Report: 2019-20 Review and 2020-21 Outlook (Aither, 2020); https://aither.com.au/wp-content/uploads/2020/08/2020-Water-Markets-Report.pdf
Grafton, R. Q. & Wheeler, S. A. Economics of water recovery in the Murray–Darling Basin, Australia. Annu. Rev. Resour. Econ. 10 , 487–510 (2018).
Moench, M. Water and the potential for social instability: livelihoods, migration and the building of society. Nat. Resour. Forum 26 , 195–204 (2002).
Water Markets in Australia: A Short History (National Water Commission, 2011).
Kundzewicz, Z. W. & Döll, P. Will groundwater ease freshwater stress under climate change? Hydrol. Sci. J. 54 , 665–675 (2009).
A Snapshot of the World’s Water Quality: Towards a Global Assessment (UNEP, 2016).
Summary Progress Update 2021: SDG 6 — Water and Sanitation for All (UN-Water, 2021).
GEMStat: Global Environmental Monitoring System, https://gemstat.org/ (UNEP, 2022).
Akhmouch, A. & Correia, F. N. The 12 OECD principles on water governance — when science meets policy. Util. Policy 43 , 14–20 (2016).
Lankford, B., Bakker, K., Zeitoun, M. & Conway, B. D. Water Security: Principles, Perspectives, and Practices (Routledge, 2013).
Potapov, P. et al. Global maps of cropland extent and change show accelerated cropland expansion in the twenty-first century. Nat. Food 3 , 19 (2022).
Fan, Y., Li, H. & Miguez-Macho, G. Global patterns of groundwater table depth. Science 339 , 940–943 (2013).
Download references
Author information
Authors and affiliations.
Bureau of Economic Geology, Jackson School of Geosciences, University of Texas at Austin, Austin, TX, USA
Bridget R. Scanlon, Sarah Fakhreddine, Ashraf Rateb, Robert C. Reedy & Alex Sun
Department of Civil and Environmental Engineering, Carnegie Mellon University, Pittsburgh, PA, USA
Sarah Fakhreddine
Water Systems and Global Change, Wageningen University, Wageningen, The Netherlands
Inge de Graaf
Global Institute for Water Security, National Hydrology Research Center, University of Saskatchewan, Saskatoon, Canada
Jay Famiglietti
Department of Civil Engineering, University of Victoria, Victoria, British Columbia, Canada
Tom Gleeson
Crawford School of Public Policy, Australian National University, Canberra, ACT, Australia
R. Quentin Grafton
Grupo de Estudios Ambientales, IMASL, CONICET, Universidad Nacional de San Luis, San Luis, Argentina
Esteban Jobbagy
Center for Water Resources Research, School of Agricultural, Earth and Environmental Sciences, University of KwaZulu Natal, Durban, South Africa
Seifu Kebede
UK Meteorological Office, Exeter, UK
Seshagiri Rao Kolusu
Leonard Konikow Hydrogeologist, Reston, VA, USA
Leonard F. Konikow
Department of Hydraulic Engineering, Tsinghua University, Beijing, China
Department of Civil, Construction and Environmental Engineering, University of Alabama, Tuscaloosa, AL, USA
Mesfin Mekonnen
Institute of Physical Geography, Goethe University Frankfurt, Frankfurt am Main, Frankfurt, Germany
Hannes Müller Schmied
Senckenberg Leibniz Biodiversity and Climate Research Centre (SBiK-F), Frankfurt am Main, Frankfurt, Germany
School of Environmental Science and Engineering, Indian Institute of Technology Kharagpur, Kharagpur, India
Abhijit Mukherjee
British Geological Survey, Lyell Centre, Edinburgh, UK
Alan MacDonald
Institute for Risk and Disaster Reduction, University College London, London, UK
Mohammad Shamsudduha
National Centre for Groundwater Research and Training (NCGRT), College of Science and Engineering, Flinders University, Adelaide, South Australia, Australia
Craig T. Simmons
Department of Geography, University College London, London, UK
Richard G. Taylor
Water Cycle Innovation Ltd, Johannesburg, Gauten, South Africa
Karen G. Villholth
Environmental Sciences Initiative, Advanced Science Research Center at the CUNY Graduate Center, New York, NY, USA
Charles J. Vörösmarty
School of Environmental Science and Engineering, Southern University of Science and Technology, Shenzhen, China
Chunmiao Zheng
You can also search for this author in PubMed Google Scholar
Contributions
B.R.S. conceptualized the review and coordinated input. S.F. reviewed many of the topics and developed some of the figures. A.R. analysed GRACE satellite data and M.S. reviewed this output. Q.G. provided input on water economics. E.J. reviewed impacts of land-use change. S.R.K. provided data on future precipitation changes. L.F.K. provided detailed information on surface water/groundwater interactions. M.M. provided data on water trade. C.J.V. provided input on green and grey solutions. All authors reviewed the paper and provided edits.
Corresponding author
Correspondence to Bridget R. Scanlon .
Ethics declarations
Competing interests.
The authors declare no competing interests.
Peer review
Peer review information.
Nature Reviews Earth & Environment thanks Helen Dahlke, Diana Allen, who co-reviewed with Aspen Anderson, and the other, anonymous, reviewer(s) for their contribution to the peer review of this work.
Additional information
Publisher’s note Springer Nature remains neutral with regard to jurisdictional claims in published maps and institutional affiliations.
Supplementary information
Supplementary information, supplementary tables , rights and permissions.
Springer Nature or its licensor (e.g. a society or other partner) holds exclusive rights to this article under a publishing agreement with the author(s) or other rightsholder(s); author self-archiving of the accepted manuscript version of this article is solely governed by the terms of such publishing agreement and applicable law.
Reprints and permissions
About this article
Cite this article.
Scanlon, B.R., Fakhreddine, S., Rateb, A. et al. Global water resources and the role of groundwater in a resilient water future. Nat Rev Earth Environ 4 , 87–101 (2023). https://doi.org/10.1038/s43017-022-00378-6
Download citation
Accepted : 17 November 2022
Published : 31 January 2023
Issue Date : February 2023
DOI : https://doi.org/10.1038/s43017-022-00378-6
Share this article
Anyone you share the following link with will be able to read this content:
Sorry, a shareable link is not currently available for this article.
Provided by the Springer Nature SharedIt content-sharing initiative
This article is cited by
Rapid groundwater decline and some cases of recovery in aquifers globally.
- Scott Jasechko
- Hansjörg Seybold
- James W. Kirchner
Nature (2024)
Winter snow deficit was a harbinger of summer 2022 socio-hydrologic drought in the Po Basin, Italy
- Francesco Avanzi
- Francesca Munerol
- Luca Ferraris
Communications Earth & Environment (2024)
Multi-disciplinary strategy to optimize irrigation efficiency in irrigated agriculture
- Ayman Batisha
Scientific Reports (2024)
Development of Groundwater Levels Dataset for Chile since 1970
- Héctor Leopoldo Venegas-Quiñones
- Rodrigo Valdés-Pineda
- Ty P. A. Ferré
Scientific Data (2024)
Comparative life cycle assessment of environmental impacts and economic feasibility of tomato cultivation systems in northern plains of India
- Rohit Kumar
- Arvind Bhardwaj
- Kanhu Charan Pattnayak
Quick links
- Explore articles by subject
- Guide to authors
- Editorial policies
Sign up for the Nature Briefing: Anthropocene newsletter — what matters in anthropocene research, free to your inbox weekly.

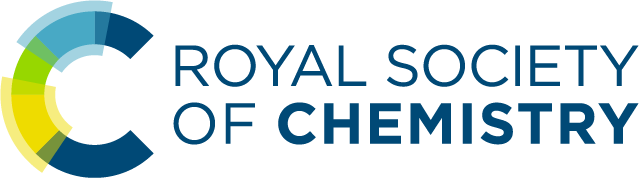
Journals, books & databases
- Our journals
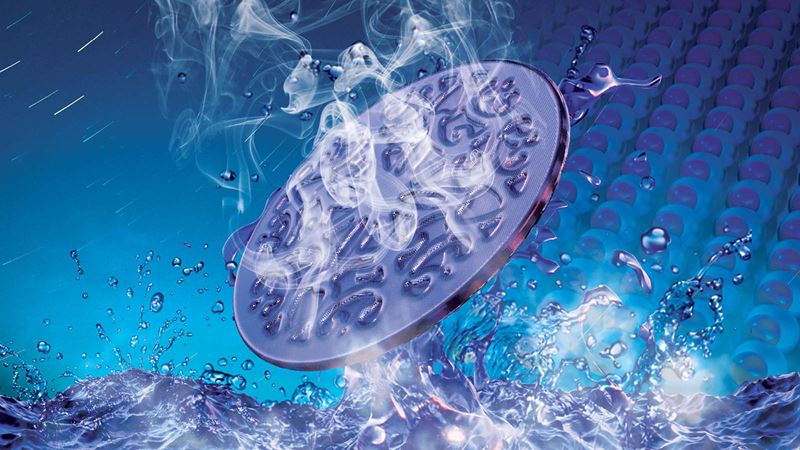
Environmental Science: Water Research & Technology
Innovation for sustainable water
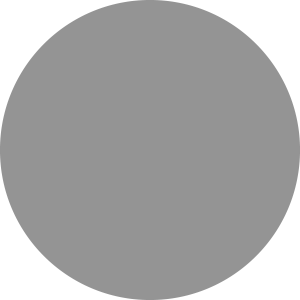
You can find details about how to access information remotely in this step-by-step guide . The guide will also help if for any reason you have difficulty accessing the content you want.
What would you like to know about this journal?
Environmental Science: Water Research & Technology is a Transformative Journal, and Plan S compliant
Impact factor: 5.0*
Time to first decision (all decisions): 14.0 days**
Time to first decision (peer reviewed only): 52.0 days***
Editor-in-Chief: Graham Gagnon
Open access publishing options available
Read this journal
Submit an article
Sign up for regular email alerts
View all journal metrics
Publish open access
Meet the team
Read our latest themed issues Urban stormwater management Data-intensive water systems management and operation Polymers in liquid formulations Drinking water oxidation and disinfection processes
Journal scope
Environmental Science: Water Research & Technology seeks to showcase high quality research about fundamental science, innovative technologies, and management practices that promote sustainable water.
The journal aims to provide a comprehensive and relevant forum that unites the diverse communities and disciplines conducting water research relevant to engineered systems and the built environment. This includes fundamental science geared toward understanding physical, chemical, and biological phenomena in these systems as well as applied research focused on the development and optimisation of engineered treatment, management, and supply strategies.
Papers must report a significant advance in the theory, fundamental understanding, practice or application of water research, management, engineering or technology, within the following areas:
- Treatment and fate of chemical and microbial contaminants, including emerging contaminants
- Water distribution and wastewater collection
- Green infrastructure
- Stormwater management and treatment
- Potable reuse
- Residue management
- Sustainability analysis and design, including life cycle assessment studies
- Municipal and industrial wastewater treatment and resource recovery
- Drinking water treatment
- Water policy and regulation
- Applications of new water technologies*
- Water, sanitation and hygiene (WASH)
- Water-energy nexus
- Simulation and data science applications to engineered water systems
- Environmental remediation of soil, sediment, and groundwater
- Impacts of climate change on engineered water systems
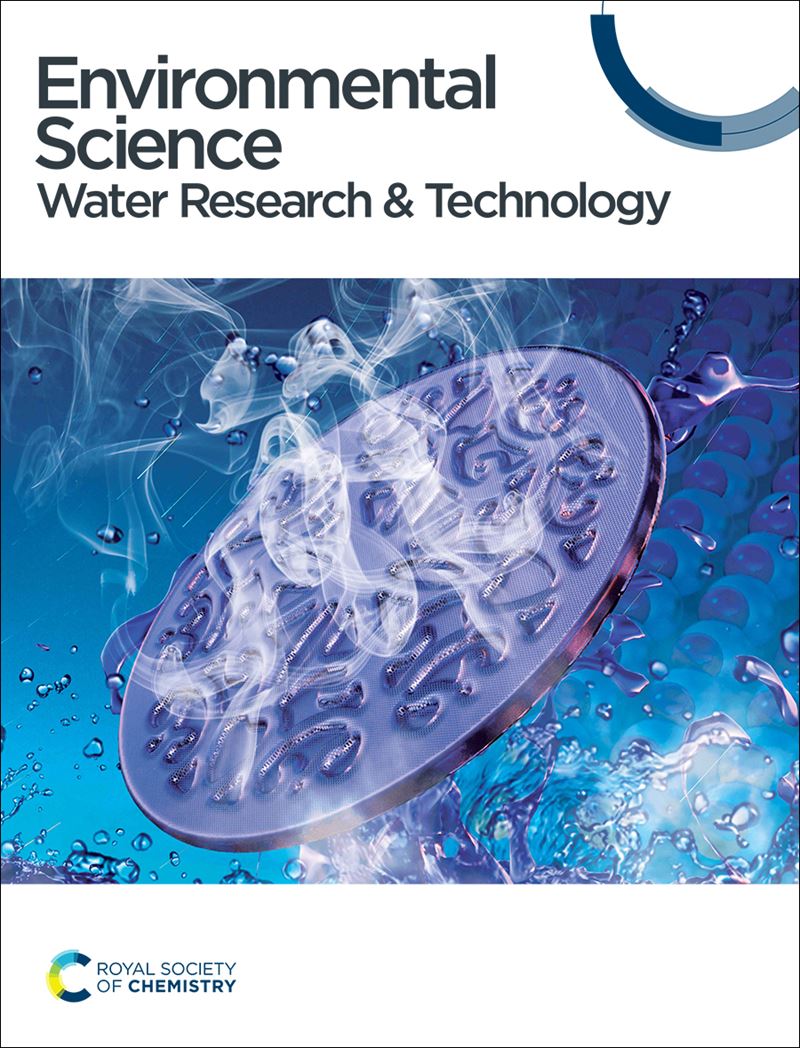
The journal places special focus on issues associated with water sustainability, as well as research that may lead to more secure, resilient and reliable water supplies. And it welcomes inter- and multidisciplinary work contributing to any of the above developments that are likely to be of interest to the broad community that the Journal addresses.
Manuscripts should be written to be accessible to scientists and engineers in all disciplines associated with the Journal.
All manuscripts must highlight their novel features and explain the significance of the work relative to related studies in their field as well as the likely impact on relevant water communities in the industry, government or academia.
*Please see the below expandable section for specific guidance regarding this area of our scope.
Measurement advances and analysis: these papers are encouraged and must clearly focus on the relevance of the work to engineered water systems and clearly explain the implications of the analysis or observations for sustainable water management. Papers dealing only with analysis, analytical method development or that simply report measured concentrations of target analytes (for example, occurrence and effluent concentrations of novel pollutant classes) will not be considered for publication.
Modeling: papers that lack appropriate validation through either experimental data or available and reliable datasets will not be considered for publication.
New materials or technologies for water treatment: emphasis must be placed on one of the following:
- Developing a fundamental understanding of the underlying mechanisms integral to technology performance
- Demonstrating how the practical application of the technology advances the field and improves upon existing treatment options
Papers in this area are strongly discouraged from focusing solely on technology demonstrations in model systems with model pollutant targets. Rather, they are encouraged to consider performance in complex (that is, environmentally relevant) systems and performance metrics (for example, efficacy across multiple pollutant targets, longevity, regeneration during application, and sustainability assessment) most relevant to real world application.
Technology papers: we will not consider papers that focus solely on any of the following:
- Heavily focused on material synthesis and characterisation (such as nanomaterial catalysts)
- Consider only the removal of highly idealised targets (such as dyes)
- Work exclusively in clean laboratory systems
- Do not demonstrate innovation that advances the treatment field, or develops a technology without a clear and viable pathway to full scale implementation
Sustainability assessments: papers that cover, for example, life cycle assessment or life cycle cost analysis, of water-related technologies and systems must emphasize the fundamental insight into the factors governing technology or system performance. Papers are strongly discouraged from solely reporting absolute or comparative assessments of technologies/systems without uncovering novel insight or identifying critical barriers to sustainability.
These guidelines will be used by our Associate editors and reviewers to assess the significance of each submitted manuscript.
See who's on the team
Meet Environmental Science: Water Research & Technology Editor-in-Chief and board members.
Editor-in-chief
Graham Gagnon , Dalhousie University, Canada
Associate editors
Sebastià Puig Broch , Universitat de Girona, Spain
Wenhai Chu , Tongji University, China
Ning Dai , University at Buffalo, USA
Lauren Stadler , Rice University, USA
Liu Ye , The University of Queensland, Australia
Editorial board members
Takahiro Fujioka , Nagasaki University, Japan
Branko Kerkez , University of Michigan, USA
Jeonghwan Kim , Inha University, South Korea
Linda Lawton , Robert Gordon University, UK
Luca Vezzaro , Technical University of Denmark, Denmark
Eveline Volcke , Ghent University, Belgium
Federico Aulenta , National Research Council, Italy
Nicholas Ashbolt , University of Alberta, Canada
Tom Bond , University of Surrey, UK
Joby Boxall , The University of Sheffield, UK
Kartik Chandran , Columbia University in the City of New York, USA
Amy Childress , University of Southern California, USA
David Cwiertny , University of Iowa
Joel Ducoste , North Carolina State University, USA
Marc Edwards , Virginia Tech, USA
Jingyun Fang , Sun Yat-sen University, China
Maria Jose Farre , Catalan Institute for Water Research, Spain
Yujie Feng , Harbin Institute of Technology, China
Kathrin Fenner , Swiss Federal Institute of Aquatic Science and Technology, Eawag, Switzerland
Ramesh Goel , University of Utah, USA
Ola Gomaa , National Center for Radiation Research and Technology, Egypt
Chris Gordon , University of Ghana, Ghana
April Gu , Cornell University, USA
Jochen Hack , TU Darmstadt, Germany
Zhen "Jason" He , Washington University in St. Louis, USA
Xia Huang , Tsinghua University, China
Cynthia Joll , Curtin University, Australia
Tamar Kohn , École Polytechnique Fédérale de Lausanne, EPFL, Switzerland
Peng Liang , Tsinghua University, China
Irene Lo , Hong Kong University of Science and Technology, Hong Kong
Julie Minton , WateReuse Foundation, USA
Vincenzo Naddeo , University of Salerno, Italy
Indumathi M Nambi , Indian Institute of Technology Madras, India
Long Ngheim , University of Technology Sydney, Australia
Paige Novak , University of Minnesota, USA
Yong Sik Ok , Korea University, South Korea
Ligy Philip , Indian Institute of Technology Madras, India
Thalappil Pradeep , Indian Institute of Technology Madras, India
Zhiyong "Jason" Ren , Princeton University, USA
Peter Robertson , Queen's University Belfast, UK
Michael Templeton , Imperial College London, UK
Kai Udert , Swiss Federal Institute of Aquatic Science and Technology, Switzerland
Subramanyan Vasudevan , CSIR-Central Electrochemical Research Institute, India
Xin Wang , Nankai University, China
David Weissbrodt , TU Delft, The Netherlands
Krista Wigginton , University of Michigan, USA
Di Wu , Ghent University, South Korea
Defeng Xing , Harbin Institute of Technology, China
Jeyong Yoon , Seoul National University, South Korea
Neil Scriven , Executive Editor
Grace Thoburn , Deputy Editor
Nour Tanbouza , Development Editor
Claire Darby , Editorial Production Manager, ORCID 0000-0003-3059-6020
Emma Carlisle, Publishing Editor
Hannah Hamilton , Publishing Editor
Ephraim Otumudia , Publishing Editor
Irene Sanchez Molina Santos , Publishing Editor
Michael Spencelayh , Publishing Editor
Callum Woof , Publishing Editor
Lauren Yarrow-Wright , Publishing Editor
Kate Bandoo , Editorial Assistant
Linda Warncke , Publishing Assistant
Sam Keltie , Publisher, Journals, ORCID 0000-0002-9369-8414
Article types
Environmental Science: Water Research & Technology publishes:
Communications
Full papers, perspectives, critical reviews, frontier reviews, tutorial reviews, comments and replies.
Reviews & Perspectives are normally invited, however suggestions for timely Reviews are very welcome. Interested authors should contact the Editorial Office at [email protected] with an abstract or brief synopsis of their intended Review.
These must report preliminary research findings that are novel and original, of immediate interest and are likely to have a high impact on the Environmental Science: Water Research & Technology community. Authors must provide a short paragraph explaining why their work justifies rapid publication as a communication.
Original research papers on any of the subjects outlined in the scope section and related areas are encouraged and welcomed. All papers should give due attention to overcoming limitations and to underlying principles. All contributions will be judged on the following four criteria. 1. Novelty and insight 2. Quality of scientific work and content 3. Clarity of objectives and aims of the work 4. Appropriateness of length to content of new science
These may be articles providing a personal view of part of one discipline associated with Environmental Science: Water Research & Technology or a philosophical look at a topic of relevance. Alternatively, Perspectives may be historical articles covering a particular subject area or the development of particular legislation, technologies, methodologies or other subjects within the scope of the journal.
Critical reviews must be a critical evaluation of the existing state of knowledge on a particular facet of water research or water technologies as they affect environmental science. They should be timely and provide insights based on existing literature. They should be of general interest to the journal's wide readership.
All Critical reviews undergo a rigorous and full peer review procedure, in the same way as regular research papers. Authors are encouraged to identify areas in the field where further developments are imminent or of urgent need, and any areas that may be of significance to the community in general. Critical reviews should not contain any unpublished original research.
These are shorter, more focused versions of Critical reviews on a well-defined, specific topic area covering approximately the last two-three years. Articles should cover only the most interesting/significant developments in that specific subject area.
The article should be highly critical and selective in referencing published work. One or two paragraphs of speculation about possible future developments may also be appropriate in the conclusion section.
Frontier reviews may also cover techniques/technologies that are too new for a Critical review or may address a subset of technologies available for a given area of research within the journal scope.
Frontier reviews should not contain unpublished original research.
Tutorial reviews should provide an introduction and overview of an important topic of relevance to the journal readership. The topic should be of relevance to both researchers who are new to the field as well as experts and provide a good introduction to the development of a subject, its current state and indications of future directions the field is expected to take. Tutorial reviews should not contain unpublished original research.
Comments and Replies are a medium for the discussion and exchange of scientific opinions between authors and readers concerning material published in Environmental Science: Water Research & Technology.
For publication, a Comment should present an alternative analysis of and/or new insight into the previously published material. Any Reply should further the discussion presented in the original article and the Comment. Comments and Replies that contain any form of personal attack are not suitable for publication.
Comments that are acceptable for publication will be forwarded to the authors of the work being discussed, and these authors will be given the opportunity to submit a Reply. The Comment and Reply will both be subject to rigorous peer review in consultation with the journal’s Editorial Board where appropriate. The Comment and Reply will be published together.
Journal specific guidelines
See a summary of ESWRT’s journal-specific guidelines . More details are also provided below.
Use of RSC template
There are no submission specifics regarding formatting; use of Royal Society of Chemistry template is not required. Bibliographies should be formatted according to the following Endnote and Zotero style files to include the cited article’s title.
Authors are encouraged to include line numbering in submitted manuscripts. Although there is no page limit for Full papers, appropriateness of length to content of new science will be taken into consideration by reviewers.
Water Impact Statement
All submitted manuscripts must include a 'Water Impact Statement' (60 words maximum; approximately three sentences) that clearly states in plain language the broad-scale implications and real-world relevance of the work. True potential for immediate real-world impact may be subject to further study, but the pathways towards achieving that impact in future should at least be envisioned and explained.
Read Professor Michael Templeton’s Editorial Perspective “ Achieving real-world impact ” for further discussion on expectations for the journal.
Authors should use this statement to show that they have given serious consideration as to how their work addresses current challenges related to water sustainability in a realistic sense. This statement will be carefully considered by the editors and the reviewers and will help ascertain the relevance of the article for a broad audience. Absence of potential for real-world impact is reason for rejection. If the manuscript is accepted this statement will be included in the published article. Please note that manuscripts without this statement will not be peer-reviewed.
Double-anonymised peer review option
Environmental Science: Water Research & Technology is now offering authors the option of double-anonymised peer review. Both single- and double-anonymised peer review are now available to authors.
- Single-anonymised peer review - where reviewers are anonymous but author names and affiliations are known to reviewers. (This is the traditional peer review model used on Environmental Science: Water Research & Technology)
- Double-anonymised peer review - where authors and reviewers' identities are concealed from each other.
Guidelines for authors and reviewers can be found here
Organisation of material
An article should have a short, straightforward title directed at the general reader. Lengthy systematic names and complicated and numerous chemical formulae should therefore be avoided where possible. The use of non-standard abbreviations and symbols in a title is not encouraged. Please bear in mind that readers increasingly use search engines to find literature; recognisable, key words should be included in the title where possible, to maximise the impact and discoverability of your work. Brevity in a title, though desirable, should be balanced against its accuracy and usefulness.
The use of series titles and part numbers in titles of papers is discouraged. Instead these can be included as a footnote to the first page together with a reference (reference 1) to the preceding part. When the preceding part has been submitted to a Royal Society of Chemistry journal but is not yet published, the paper reference number should be given.
Author names
Full names for all the authors of an article should be given. To give due acknowledgement to all workers contributing to the work, those who have contributed significantly to the research should be listed as co-authors. Authors who contributed equally can be noted with a Footnote and referenced with a symbol.
On submission of the manuscript, the corresponding author attests to the fact that those named as co-authors have agreed to its submission for publication and accepts the responsibility for having properly included all (and only) co- authors. If there are more than 10 co-authors on the manuscript, the corresponding author should provide a statement to specify the contribution of each co-author. The corresponding author signs a copyright licence on behalf of all the authors.
Table of contents entry
This entry should include a colour image (no larger than 8 cm wide x 4 cm high), and 20-30 words of text that highlight the novel aspects of your work. Graphics should be as clear as possible; simple schematic diagrams or reaction schemes are preferred to ORTEP- style crystal structure depictions and complicated graphs, for example. The graphic used in the table of contents entry need not necessarily appear in the article itself. Authors should bear in mind the final size of any lettering on the graphic. For examples, please see the online version of the journal.
Every paper must be accompanied by a summary (50-250 words) setting out briefly and clearly the main objects and results of the work; it should give the reader a clear idea of what has been achieved. The summary should be essentially independent of the main text; however, names, partial names or linear formulae of compounds may be accompanied by the numbers referring to the corresponding displayed formulae in the body of the text.
Please bear in mind that readers increasingly use search engines to find literature; recognisable, searchable terms and key words should be included in the abstract to enable readers to more effectively find your paper. The abstract should aim to address the following questions.
- What is the problem or research question being addressed?
- What experimental approach was used to address the problem or question?
- What key data and results were obtained?
- What conclusions can be drawn from the experimental results?
- What are the broader implications for the study with respect to water sustainability?
Water Impact Statement
Authors must provide a 'Water Impact Statement' (60 words maximum) that clearly highlights the broad-scale implications and real-world relevance of the work. This statement should be different from the abstract and must set the work in broader context with regards to water sustainability. True potential for immediate real-world impact may be subject to further study, but the pathways towards achieving that impact in future should at least be envisioned and explained in this statement.
When composing your Water Impact Statement, please consider the following points:
1.What is the problem? 2.Why is it important? 3.How does this translate to real-world applications/scenarios? 4.How can this be generalised? 5.Why is this work significant for ensuring sustainable water resources?
This statement will be seen by the reviewers and will help ascertain the relevance of the article for a broad but technical audience. Authors should use it to show that they have given serious consideration to the impact of their presented study. Absence of potential for real-world impact is reason for rejection. If the paper is accepted this statement will also be published. Please note that papers cannot be peer-reviewed without this statement.
Introduction
This should give clearly and briefly, with relevant references, both the nature of the problem under investigation and its background.
Descriptions of methods and/or experiments should be given in detail sufficient to enable experienced experimental workers to repeat them. Standard techniques and methods used throughout the work should be stated at the beginning of the section. Apparatus should be described only if it is non-standard; commercially available instruments are referred to by their stock numbers (for example, Perkin-Elmer 457 or Varian HA-100 spectrometers). The accuracy of primary measurements should be stated. In general there is no need to report unsuccessful experiments. Authors are encouraged to make use of electronic supplementary information (ESI) for lengthy synthetic sections. Any unusual hazards inherent in the use of chemicals, procedures or equipment in the investigation should be clearly identified. In cases where a study involves the use of live animals or human subjects, the author should include a statement that all experiments were performed in compliance with the relevant laws and institutional guidelines, and also state the institutional committee(s) that have approved the experiments. They should also include a statement that informed consent was obtained for any experimentation with human subjects. Referees may be asked to comment specifically on any cases in which concerns arise.
Results and discussion
It is usual for the results to be presented first, followed by a discussion of their significance. Only strictly relevant results should be presented and figures, tables, and equations should be used for purposes of clarity and brevity. The use of flow diagrams and reaction schemes is encouraged. Data must not be reproduced in more than one form - for example, in both figures and tables, without good reason.
This is for interpretation and to highlight the novelty and significance of the work. Authors are encouraged to discuss the real world relevance of the work reported and how it promotes water sustainability. The conclusions should not summarise information already present in the text or abstract.
Acknowledgements
Contributors other than co-authors may be acknowledged in a separate paragraph at the end of the paper; acknowledgements should be as brief as possible. All sources of funding should be declared.
Bibliographic references and notes
These should be listed at the end of the manuscript in numerical order. We encourage the citation of primary research over review articles, where appropriate, in order to give credit to those who first reported a finding. Find out more about our commitments to the principles of San Francisco Declaration on Research Assessment (DORA).
Bibliographic details should be cited in the order: year, volume , page, and must include the article title. For example: Lukas Mustajärvi, Ann-Kristin Eriksson-Wiklund, Elena Gorokhova, Annika Jahnke and Anna Sobek, Transferring mixtures of chemicals from sediment to a bioassay using silicone-based passive sampling and dosing, Environ. Sci.: Processes Impacts , 2017, 19 , 1404-1413. See Endnote style files . For Zotero, please use the Royal Society of Chemistry (with titles) template.
Bibliographic reference to the source of statements in the text is made by use of superior numerals at the appropriate place (for example, Wittig3). The reference numbers should be cited in the correct sequence through the text (including those in tables and figure captions, numbered according to where the table or figure is designated to appear). Please do not use Harvard style for references.
The references themselves are given at the end of the final printed text along with any notes. The names and initials of all authors are always given in the reference; they must not be replaced by the phrase et al . This does not prevent some, or all, of the names being mentioned at their first citation in the cursive text; initials are not necessary in the text. Notes or footnotes may be used to present material that, if included in the body of the text, would disrupt the flow of the argument but which is, nevertheless, of importance in qualifying or amplifying the textual material. Footnotes are referred to with the following symbols: †, ‡, §, ¶, ║etc.
Alternatively the information may be included as Notes (end-notes) to appear in the Notes/references section of the manuscript. Notes should be numbered using the same numbering system as the bibliographic references.
Journals The style of journal abbreviations to be used in RSC publications is that defined in Chemical Abstracts Service Source Index (CASSI) (http://www.cas.org/expertise/cascontent/caplus/corejournals.html).
Bibliographic details should be cited in the order: year, volume , page. Where page numbers are not yet known, articles should be cited by DOI (Digital Object Identifier) - for example, T. J. Hebden, R. R. Schrock, M. K. Takase and P. Müller, Chem. Commun ., 2012, DOI: 10.1039/C2CC17634C.
Books J. Barker, in Catalyst Deactivation , ed. B. Delmon and C. Froment, Elsevier, Amsterdam, 2nd edn., 1987, vol. 1, ch. 4, pp. 253-255.
Patents Br. Pat ., 357 450, 1986. US Pat ., 1 171 230, 1990.
Reports and bulletins, etc R. A. Allen, D. B. Smith and J. E. Hiscott, Radioisotope Data , UKAEA Research Group Report AERE-R 2938, H.M.S.O., London, 1961.
Material presented at meetings H. C. Freeman, Proceedings of the 21st International Conference on Coordination Chemistry, Toulouse, 1980.
Theses A. D. Mount, Ph.D. Thesis, University of London, 1977.
Reference to unpublished material For material presented at a meeting, congress or before a Society, etc., but not published, the following form is used: A. R. Jones, presented in part at the 28th Congress of the International Union of Pure and Applied Chemistry, Vancouver, August, 1981.
For material accepted for publication, but not yet published, the following forms are used.
- A. R. Jones, Dalton Trans. , 2003, DOI: 10.1039/manuscript number, for RSC journals
- A. R. Jones, Angew. Chem ., in press, for non-RSC journals
If DOI numbers are known these should be cited in the form recommended by the publisher.
For material submitted for publication but not yet accepted the following form is used.
- A. R. Jones, Angew. Chem ., submitted.
For personal communications the following is used.
- G. B. Ball, personal communication.
If material is to be published but has yet to be submitted the following form is used.
- G. B. Ball, unpublished work.
Reference to unpublished work should not be made without the permission of those by whom the work was performed.
Software F James, AIM2000, version 1.0, University of Applied Sciences, Bielefeld, Germany, 2000. T Bellander, M Lewne and B Brunekreef, GAUSSIAN 3 (Revision B.05), Gaussian Inc., Pittsburgh, PA, 2003.
Online resources (including databases) Please note the most important information to include is the URL and the data accessed.
- The Merck Index Online, http://www.rsc.org/Merck-lndex/monograph/mono1500000841, (accessed October 2013).
- ChemSpider, http://www.chemspider.com/Chemicai-Structure.1906.html, (accessed June 2011).
arXiv references V. Krstic and M. Glerup, 2006, arXiv:cond-mat/0601513.
Figures & schemes
Preparation of graphics.
Artwork should be submitted at its final size so that reduction is not required. The appearance of graphics is the responsibility of the author.
- Graphics should fit within either single column (8.3 cm) or double column (17.1 cm) width, and must be no longer than 23.3 cm.
- Graphical abstracts should be no larger than 8 x 4 cm.
- Schemes and structures should be drawn to make best use of single and double column widths.
Colour figures
Colour figure reproduction is provided free of charge both online and in print.
Journal covers
Authors who wish to have their artwork featured on a journal cover should contact the editorial office of the journal to which the article is being submitted. A contribution to the additional production costs will be requested.
Use of such artwork is at the editor's discretion; the editor's decision is final. Examples of previous journal covers can be viewed via the journal homepage.
Electronic supplementary information
The journal's electronic supplementary information (ESI) service is a free facility that enables authors to enhance and increase the impact of their articles. Authors are encouraged to make the most of the benefits of publishing supplementary information in electronic form. Such data can take full advantage of the electronic medium, allowing use of 3D molecular models and movies. Authors can also improve the readability of their articles by placing appropriate material, such as repetitive experimental details and bulky data, as ESI. All information published as ESI is also fully archived. When preparing their ESI data files, authors should keep in mind the following points.
- Supplementary data is peer-reviewed, and should therefore be included with the original submission.
- ESI files are published 'as is'; editorial staff will not usually edit the data for style or content.
- Data is useful only if readers can access it; use common file formats.
- Large files may prove difficult for users to download and access.
Text and graphics
The preferred format for ESI comprising text and graphics is Microsoft Word. Publishing staff will convert Word files to PDF before publication, as this format can be accessed easily and reliably on most computing platforms using the freely available Adobe Acrobat Reader. If other formats are submitted they will also usually be converted to PDF files prior to publication.
Multimedia files
We welcome submission of multimedia files (including videos and animations) alongside articles for publication. Videos are an excellent medium to present elements of your work that can be difficult to communicate only in words. Please note that any videos of general interest are shared with the wider community via the RSC Journals YouTube channel. Please notify the editorial team if you prefer for your video(s) not to be uploaded to YouTube. If you submit a multimedia file alongside your paper, please refer to it within your paper to draw it to the reader’s attention. Also please see the section on submitting multimedia files
Format Acceptable formats for video or animation clips are listed below.
Please minimise file sizes where you can, by considering the following points.
- The recommended maximum frame size is 640 x 480 pixels.
- Our recommended maximum file size is 5 Mb.
- Many packages output 30 frames per second (fps) as standard, but it's possible to specify a lower frame rate; this may not noticeably affect the quality of your video but will reduce the file size.
- Use a 256 colour palette, if that is suitable for the presentation of the material.
Please consider the use of lower specifications for all these points if the material can still be represented clearly.
If your video is very short (that is, several seconds long) then it is recommended that you loop it and repeat a few times to provide a more detailed view.
Submitting multimedia files Upload your video online, together with your manuscript under the category 'electronic supplementary material' and please supply the following.
- A clear file name for your video.
- A short descriptive title for the video, which can be used when uploading the video onto a streaming channel.
- A video legend of approximately 30 words long; this caption must be provided to aid discoverability.
- Five to 10 keywords that can be used to tag the video; the more accurate the tags are the better discoverability videos will have.
Copies of any relevant 'in press' references
Manuscripts should be submitted with copies of any ‘in press’ articles referenced.
Open access publishing options
Environmental Science: Water Research & Technology is a hybrid (transformative) journal and gives authors the choice of publishing their research either via the traditional subscription-based model or instead by choosing our gold open access option. Find out more about our Transformative Journals. which are Plan S compliant .
Gold open access
For authors who want to publish their article gold open access , Environmental Science: Water Research & Technology charges an article processing charge (APC) of £2,750 (+ any applicable tax). Our APC is all-inclusive and makes your article freely available online immediately, permanently, and includes your choice of Creative Commons licence (CC BY or CC BY-NC) at no extra cost. It is not a submission charge, so you only pay if your article is accepted for publication.
Learn more about publishing open access .
Read & Publish
If your institution has a Read & Publish agreement in place with the Royal Society of Chemistry, APCs for gold open access publishing in Environmental Science: Water Research & Technology may already be covered.
Use our journal finder to check if your institution has an open access agreement with us.
Please use your official institutional email address to submit your manuscript and check you are assigned as the corresponding author; this helps us to identify if you are eligible for Read & Publish or other APC discounts.
Traditional subscription model
Authors can also publish in Environmental Science: Water Research & Technology via the traditional subscription model without needing to pay an APC. Articles published via this route are available to institutions and individuals who subscribe to the journal. Our standard licence allows you to make the accepted manuscript of your article freely available after a 12-month embargo period. This is known as the green route to open access.
Learn more about green open access .
Subscription information
Online only 2024: ISSN: 2053-1419, £2,031 / $3,352
*2022 Journal Citation Reports (Clarivate Analytics, 2023)
**The median time from submission to first decision including manuscripts rejected without peer review from the previous calendar year
***The median time from submission to first decision for peer-reviewed manuscripts from the previous calendar year
Advertisement
Water Environment Research
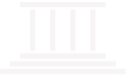
Subject Area and Category
- Ecological Modeling
- Environmental Chemistry
- Waste Management and Disposal
- Water Science and Technology
Publication type
10614303, 15547531
Information
How to publish in this journal
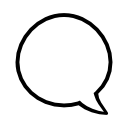
The set of journals have been ranked according to their SJR and divided into four equal groups, four quartiles. Q1 (green) comprises the quarter of the journals with the highest values, Q2 (yellow) the second highest values, Q3 (orange) the third highest values and Q4 (red) the lowest values.
The SJR is a size-independent prestige indicator that ranks journals by their 'average prestige per article'. It is based on the idea that 'all citations are not created equal'. SJR is a measure of scientific influence of journals that accounts for both the number of citations received by a journal and the importance or prestige of the journals where such citations come from It measures the scientific influence of the average article in a journal, it expresses how central to the global scientific discussion an average article of the journal is.
Evolution of the number of published documents. All types of documents are considered, including citable and non citable documents.
This indicator counts the number of citations received by documents from a journal and divides them by the total number of documents published in that journal. The chart shows the evolution of the average number of times documents published in a journal in the past two, three and four years have been cited in the current year. The two years line is equivalent to journal impact factor ™ (Thomson Reuters) metric.
Evolution of the total number of citations and journal's self-citations received by a journal's published documents during the three previous years. Journal Self-citation is defined as the number of citation from a journal citing article to articles published by the same journal.
Evolution of the number of total citation per document and external citation per document (i.e. journal self-citations removed) received by a journal's published documents during the three previous years. External citations are calculated by subtracting the number of self-citations from the total number of citations received by the journal’s documents.
International Collaboration accounts for the articles that have been produced by researchers from several countries. The chart shows the ratio of a journal's documents signed by researchers from more than one country; that is including more than one country address.
Not every article in a journal is considered primary research and therefore "citable", this chart shows the ratio of a journal's articles including substantial research (research articles, conference papers and reviews) in three year windows vs. those documents other than research articles, reviews and conference papers.
Ratio of a journal's items, grouped in three years windows, that have been cited at least once vs. those not cited during the following year.
Evolution of the percentage of female authors.
Evolution of the number of documents cited by public policy documents according to Overton database.
Evoution of the number of documents related to Sustainable Development Goals defined by United Nations. Available from 2018 onwards.
Leave a comment
Name * Required
Email (will not be published) * Required
* Required Cancel
The users of Scimago Journal & Country Rank have the possibility to dialogue through comments linked to a specific journal. The purpose is to have a forum in which general doubts about the processes of publication in the journal, experiences and other issues derived from the publication of papers are resolved. For topics on particular articles, maintain the dialogue through the usual channels with your editor.
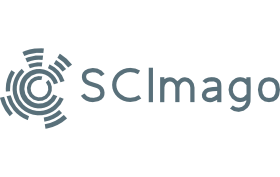
Follow us on @ScimagoJR Scimago Lab , Copyright 2007-2024. Data Source: Scopus®

Cookie settings
Cookie Policy
Legal Notice
Privacy Policy
advancing the science of water ®
Advance notice.
Upcoming request for proposals
Quantifying Energy Optimization
Developing a framework for reporting
Reducing Legionella Levels
Service lines and premise plumbing
Occurrence of PFAS Compounds
In US wastewater treatment plants
- 02 Energy Optimization
- 03 Biofilms
Topics of Focus
In the United States alone, billions of gallons of water are treated each day at water resource recovery facilities. Once the water is clean, a different challenge remains: determining what to do with the solids that are removed during the treatment process. The resulting mixture is often a unique semi-solid blend of organic and inorganic materials, trace elements, chemicals, and even pathogens, so there is no across the board solution for handling and processing the combinations of constituents that may be present.
Because these solids are often rich in nutrients, like nitrogen and phosphorus—which also happen to be the perfect ingredients for promoting healthy soil and plant growth—many facilities have turned to land application. Before these solids can be put to use for things like fertilizing farmland, however, they must undergo rigorous treatment to meet stringent regulations, at which point they become known as biosolids.
For more information, contact Ashwin Dhanasekar .
Characterization and Contamination Testing of Source Separated Organic Feedstocks and Slurries for Co-Digestion at Resource Recovery Facilities
Project highlights.
A key challenge with source separated organic (SSO) feedstock co-substrate is that its composition, quality, and characteristics differ between geographical locations and can change over time. This causes challenges and uncertainties for pre-treaters, substrate brokers, facilities accepting this material, operators...
The Water Research Foundation Funds 26 New Research Projects Totaling $5.9M
(Denver, CO) 05/28/24– The Water Research Foundation (WRF) is seeking volunteer participants for 26 new research projects funded through WRF's Research Priority Program . This strategic research program enables WRF...
Interview with Dr. William Tarpeh
Turning Waste into Gold with Dr. William Tarpeh A rare few people end up in the career they decided for themselves as children. More often, the question “What do you...
WRF Presents $100K Research Award To Advance Wastewater Resource Recovery
(Denver, CO) 10/11/23 – Last week, The Water Research Foundation (WRF) presented William Tarpeh, PhD, with the esteemed 2023 Paul L. Busch Award. With this $100,000 research prize, Dr. Tarpeh...
Climate Change
Climate change is altering our natural hydrologic cycle, creating uncertainty when it comes to the quality and quantity of water sources. WRF’s research on climate change covers the key areas of climate risk assessment, climate adaptation, and mitigation strategies.
Because the first step in preparing for climate change is understanding the potential and variable impacts these changes can have on water sources and treatment systems, WRF research tracks potential outcomes, considering a variety of possibilities, and provides resources and tools to help facilities identify and address risks and vulnerabilities in their operations and infrastructure.
Implementing climate change adaptation strategies will be critical as the water sector moves forward. WRF’s research in this area helps utilities create better long- and short-term adaptation plans, respond more effectively to severe weather, and improve infrastructure and operations to meet changing needs, including the production of onsite energy systems and reliable back-up power to protect critical services.
The water sector must also have a hand in mitigating the root causes of climate change. By pioneering approaches to improve energy efficiency, including process optimization, improved energy management, and the use of renewable energy, WRF is helping the water sector decrease activity that is driving these changes.
For more information, contact Harry Zhang .
Holistic Approaches to Flood Mitigation Planning and Modeling under Extreme Events and Climate Impacts
Municipalities and utilities are facing unprecedented challenges in planning for extreme precipitation and flooding events, which are occurring more frequently and unpredictably. A holistic approach to flood mitigation planning and modeling, including partnerships between stakeholders, is needed to balance competing...
One Water Cities: A Self-Assessment Framework
Municipalities play key roles in implementing One Water approaches and furthering community resilience. Read the full article.
The Water Research Foundation Honors Outstanding Water Leaders
(Denver, CO) 6/21/23 - The Water Research Foundation (WRF) announced last week that it awarded its 2023 Subscriber Impact Award to Denver Water and its 2023 Research Innovation Award to...
Cyanobacteria & Cyanotoxins
Aquatic microscopic algae and cyanobacteria (formerly known as blue-green algae) occur naturally in most surface waters. However certain nutrient and temperature conditions can cause them to multiply rapidly, leading to “blooms.” Under certain conditions, some species of cyanobacteria can produce toxic secondary metabolites or cyanotoxins, which may pose health risks to humans and animals. Even when cyanobacteria are not toxic, they can produce unpleasant tastes and odors.
Cyanobacteria continue to be among the most problematic organisms in fresh water systems. Without clear guidance or consensus regulations in place, many utilities struggle with responding to cyanobacterial harmful algal bloom (cHAB) events. Since 1994, WRF has completed more than 40 research projects on these microscopic organisms and the cyanotoxins they produce, helping facilities detect, monitor, and manage these organisms—as well as communicate with the public.
For more information, contact Sydney Samples .
Refinement and Standardization of Cyanotoxin Analytical Techniques for Drinking Water
There is uncertainty relating to the screening and confirmation of cyanotoxin samples. Water utilities need robust and dependable methods to monitor cyanotoxins in source water, through the treatment process, and at the tap, as well as to make appropriate decisions...
The Water Research Foundation Funds Utility Research Projects Worth $5M in Research Value
(Denver, CO) 12/19/2023 – The Water Research Foundation (WRF) has selected twelve new projects for funding through its Tailored Collaboration Program, totaling over $5 million in research value. The projects...
PFAS Communication Guidance
Water sector professionals need to be able to communicate with their customers clearly, concisely, and consistently about per- and polyfluoroalkyl substances (PFAS). This may include information on what PFAS are...
Per- and Polyfluoroalkyl Substances
Per- and polyfluoroalkyl substances (PFAS) are man-made compounds with fluorinated carbon chains. They are resistant to heat, oil, and water, making them useful in a wide variety of products, including...
Disinfection Byproducts (DBPs)
The use of strong oxidants to disinfect water has virtually eliminated waterborne diseases like typhoid, cholera, and dysentery in developed countries. However, research has shown that chlorine interacts with natural organic matter present in water supplies to form regulated and emerging disinfection byproducts (DBPs).
To minimize the formation of regulated DBPs and comply with existing regulations, water utilities have increasingly been moving away from chlorine to use alternative disinfectants like chloramine, or installing more advanced and costly treatment processes, such as ozone or granular activated carbon to remove DBP precursors. However, while reducing the formation of halogenated DBPs, alternative oxidants have been shown to favor the formation of other DBPs (e.g., ozone producing bromate and halonitromethanes, and chloramines producing N-nitrosodimethylamine and iodinated DBPs).
For more information, contact Kenan Ozekin .

Impact of Haloacetic Acid MCL Revisions on DBP Exposure and Health Risk Reduction
The U.S. Environmental Protection Agency (EPA) is considering changes to the disinfectant and disinfection byproducts (D/DBP) rule. Specifically, there may be a shift from the currently regulated five haloacetic acids (HAA5) to nine (HAA9), which would include four additional brominated...
WRF Seeks Pre-proposals for High-Priority Utility Research
(Denver, CO) 02/15/24 – The Water Research Foundation (WRF) is now accepting pre-proposals for its matching research program, the Tailored Collaboration Program. The Tailored Collaboration Program provides an opportunity for...
The Water Research Foundation Seeks Nominations for Paul L. Busch Award
(Denver, CO) 02/08/24 – The Water Research Foundation (WRF) is now accepting nominations for the 2024 Paul L. Busch Award. The $100,000 award recognizes one outstanding individual for innovative research...
Energy Optimization
For most water facilities, energy is one of the highest costs in their operating budget. Stricter regulations are pushing facilities to use even more advanced—and energy-intensive—treatment technologies. Optimizing energy use can provide huge cost savings and numerous additional benefits, including improving air quality, protecting the environment, and bolstering energy security. WRF has published more than 100 projects that explore ways to not only optimize current energy use, but to generate power as well—setting the course for a self-sufficient water sector.
Developing a Framework for Quantifying Energy Optimization Reporting
Energy projects within the water sector are often discretionary and initiated based on projected annual energy savings metrics. The water sector lacks standard energy savings estimation procedures, as well as measurement and verification approaches and procedures that adhere to the...
Intelligent Water Systems
As with other industries, newly developed technologies drive water utilities to adapt their day-to-day operations. Water networks have been a special focus, with new instrumentation options for water production, transmission, distribution, wastewater collection, and consumer end-points coming to market. Implementing these technologies can improve the efficiency and reliability of water networks, but with myriad options, utilities need guidance on which technologies are most worthwhile and how they should be implemented.

Quantifying the Impact of Artificial Intelligence/Machine Learning-Based Approaches to Utility Performance
The purpose of this project is to survey the water industry and identify the use cases for artificial intelligence (AI) and machine learning (ML), quantify their benefits, and provide a framework for others to be able to make the same...
2024 Intelligent Water Systems Challenge
The Leaders Innovation Forum for Technology (LIFT) program, a joint effort of The Water Research Foundation (WRF) and the Water Environment Federation (WEF), is holding the sixth Intelligent Water Systems...
WRF Seeks Proposals for 22 New Research Projects Totaling $4.9M
(Denver, CO) 9/12/23 - The Water Research Foundation (WRF) is now accepting proposals for 22 research projects totaling $4.9M that will advance the science of water for communities around the...
The Water Research Foundation and Water Environment Federation Launch the Fifth Intelligent Water Systems Challenge
(Denver, CO) 02/6/23 – The Water Research Foundation and Water Environment Federation are pleased to invite teams to participate in the fifth annual Intelligent Water Systems (IWS) Challenge. As technology...
Microbes & Pathogens
Control of microbes in water systems is critical to achieving water quality and public health goals. While most microbes are not considered human pathogens, certain microbes can pose health risks or contribute undesirable tastes and odors.
Since the early 20th century, modern drinking water treatment has made great advancements in the detection, removal, and inactivation of bacteria, viruses, and protozoa. As technologies in the drinking water space continue to progress, new challenges have arisen in the form of opportunistic premise plumbing pathogens.
Wastewater and stormwater utilities also play an essential role in reducing the pathogen load to receiving waters used for recreation. Additionally, more recent advancements in water reuse, especially direct potable reuse, demand more understanding of pathogen detection, removal, and inactivation in wastewater.
For more information, contact Grace Jang (drinking water & reuse) or Lola Olabode (wastewater).
Demonstrating the Effectiveness of Flushing for Reducing the Levels of Legionella in Service Lines and Premise Plumbing
Legionella are pervasive environmental bacteria that can incidentally cause severe and sometimes fatal infections upon inhalation. Because Legionella inhabit engineered environments and proliferate in warm, stagnant premise water systems, the majority of outbreaks are associated with preventable water system maintenance...
Interview with Cheryl Norton
Cheryl Norton’s Lasting Journey with WRF and the Water Sector From leading a Water Research Foundation (WRF)- funded project right out of college, to becoming an integral member of the...
Resource Recovery
In recent decades, the wastewater sector has moved away from the idea of wastewater treatment plants as waste disposal facilities, instead envisioning these plants as water resource recovery facilities (WRRFs). WRRFs can produce clean water, recover nutrients (such as phosphorus and nitrogen), and potentially reduce fossil fuel consumption through the production and use of renewable energy.
For more information, contact Jeff Moeller .
Recent Updates
Reporting Period: November 2023 – April 2024
Reporting Period: January 1 – April 15, 2024
Reporting Period: November 2023 – April 30, 2024
Reporting Period: August 1, 2023 – March 15, 2024
Reporting Period: September 15, 2023 – March 20, 2024
Reporting Period: October 1, 2023 – December 31, 2023
Reporting Period: December 7, 2023 – March 6, 2024
Reporting Period: October 1, 2023 – April 1, 2024
Reporting Period: January 1 – March 31, 2024
Reporting Period: December 1, 2023 – March 1, 2024
Throughout the year, WRF hosts and participates in events that focus on critical water quality issues. From web seminars to research workshops, these events provide opportunities for you to learn about new research from water quality experts and to share ideas and connect with other industry professionals.
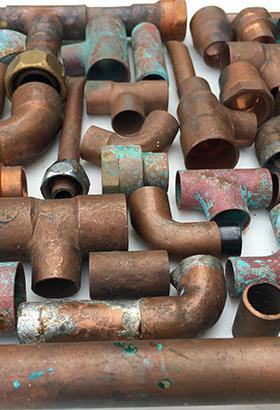
Guidance for Complying with the Lead and Copper Rule Revisions for Water Systems with No- to Low Prevalence of Lead Service Lines (LSL, LSLs)
Advances in water research.
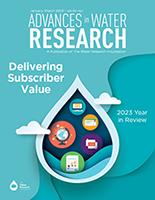
This issue highlights the essential research The Water Research Foundation delivered in 2023 thanks to the valuable contributions of our researchers, participating utilities, and countless volunteers.
Join our mailing list and receive news and updates in your inbox!

Research to uncover the impact of water use in the Colorado River Basin
The Colorado River is a lifeline for many cities and farms in the Southwest United States. It flows for about 1,448 miles before reaching the Gulf of California in Mexico and supplies water to numerous cities and farms along the way.
However, over the past 60 years, the amount of water in the Colorado River has been shrinking. In fact, in some years, the river's water has been used up completely before it reaches the gulf.
Landon Marston, assistant professor in civil and environmental engineering, teamed up with researchers from multiple universities and nongovernmental organizations to find out where the river's water goes and who uses it. This effort is informing state and federal decision-makers as they prepare to introduce new measures to bring the basin's water demands in balance with its dwindling supplies.
The research group, which includes two of Marston's graduate students, started by quantifying how much water people and businesses used from the Colorado River from 2000-19 from the records of the Bureau of Reclamation. These records serve as the basis for decision-making by local, state, and federal stakeholders concerned about the allocation of the Colorado River.
The group filled in gaps in federal records using a collection of models and data detailing crop-specific water consumption, water exported out of the basin by canals and pipelines, and evaporation from reservoirs and wetlands.
The study found that the agricultural demand for water is significantly higher than the water used by cities. The crops that need the most water are ones used for feeding cattle, such as alfalfa and hay, which are abundant in the area. The states that line the Colorado River raise roughly 14 million cattle per year.
"With water becoming scarcer and reservoir levels dropping, there is a growing need to find ways to use water more efficiently," said Marston. The reduction of water is crucial and will likely need to increase in the coming years due to competing demands and climate changes that reduce runoff into the Colorado River.
In 16 of the years analyzed, more water was taken from the river than what naturally flowed into it. To make up for the shortage, water was removed from reservoirs along the river, including Lake Mead and Lake Powell, which are among the largest reservoirs in the United States but have now become three-quarters empty.
The researchers found that the amount of water used actually varies a lot from year to year. In recent years, there has been less water used in the Lower Basin because of new rules that require more efficient use of water and cuts to the overall supply.
Another use of a significant amount of water is to support the natural environment along the river, including plants and wetlands.
"This is important because it wasn't accounted for in previous studies," said Marston. "This is not just about farms and cities. It is about protecting the river's ecosystem and ensuring a sustainable water supply for everyone in the southwestern United States."
Understanding where the water goes is crucial for making informed decisions about water use in the Colorado River Basin.
"The challenges are there," noted Marston. "But this data provides valuable information that can guide the seven states overlaying the Colorado River Basin and the federal government as they continue to negotiate how to share the dwindling water in the river."
- Drought Research
- Resource Shortage
- Environmental Policies
- World Development
- Funding Policy
- Water resources
- Water scarcity
- Anger management
- Sustainable land management
Story Source:
Materials provided by Virginia Tech . Original written by Courtney Sakry. Note: Content may be edited for style and length.
Journal Reference :
- Brian D. Richter, Gambhir Lamsal, Landon Marston, Sameer Dhakal, Laljeet Singh Sangha, Richard R. Rushforth, Dongyang Wei, Benjamin L. Ruddell, Kyle Frankel Davis, Astrid Hernandez-Cruz, Samuel Sandoval-Solis, John C. Schmidt. New water accounting reveals why the Colorado River no longer reaches the sea . Communications Earth & Environment , 2024; 5 (1) DOI: 10.1038/s43247-024-01291-0
Cite This Page :
Explore More
- More Effective Multipurpose Robots
- CO2 Conversion at a Much Larger Scale
- The Embryo Assembles Itself
- Thawing Permafrost: Not A Tipping Point
- Climate Change Was No Problem for Sharks
- Fungus Breaks Down Ocean Plastic
- Kinship and Ancestry of the Celts
- How Statin Therapy May Prevent Cancer
- Origins of 'Welsh Dragons' Exposed
- Resting Brain: Neurons Rehearse for Future
Trending Topics
Strange & offbeat.
- Other WEF Publications
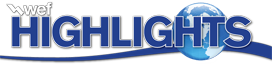
- WEF Resources & Efforts
- Learning Opps
- Laws & Regs
- Technologies
- Achievements
- Participate

WERF and WateReuse Merge To Advance Concept of One Water
June 20, 2016
Participate , Technologies
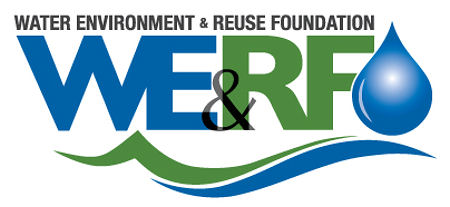
Photo courtesy of the Water Environment & Reuse Foundation (Alexandria, Va.).
The Water Environment Research Foundation and the WateReuse Research Foundation have merged to form the Water Environment & Reuse Foundation (WE&RF; Alexandria, Va.). The merger, announced May 9, brings together the organizations’ expansive portfolio of research on water, wastewater, and stormwater topics. It also reflects on the sector’s movement toward the concept of “one water,” according to a WE&RF news release.
“Bringing these two organizations together allows us to leverage our expertise to pursue an integrated approach to critical research in water,” said WE&RF Board Co-Chair Kevin Shafer, executive director of the Milwaukee Metropolitan Sewerage District, in the release. “We are now the largest one-stop-shop for research in resource recovery and reuse.”
The Water Environment Research Foundation was established in 1989 to research wastewater and stormwater. The WateReuse Research Foundation was established in 1993 to research water reuse. WE&RF will continue focusing research on resource recovery and reuse to help meet the growing demand for clean water. The nonprofit plans to identify, support, and disseminate research that enhances the quality and reliability of water for natural systems and communities with an integrated approach to resource recovery and reuse. More than 200 utilities; business, industrial, and commercial enterprises; educational institutions; and government agencies support it, the news release says.
“Our organizations shared a common goal, which is to produce research that solved real-world problems with water quality and supply,” said WE&RF Board Co-Chair Doug Owner, executive vice president and chief technical officer at Arcadis (Highlands Ranch, Colo.). “By merging, we will be able to help more communities produce a safe, reliable, locally controlled supply of water that protects the environment and supports economic growth.”
Read WE&RF Chief Executive Officer Melissa Meeker’s thoughts on the future of the water sector and merger in the WEF Highlights article, “ WERF Executive Director Melissa Meeker Discusses Water Sector Future and WERF–WRRF Merger .”
Connect with us
Visit WEF's other social media outlets.

Related Posts:
- 5 Ways to Use Social Media to Promote the Value of Water
- Apply for Water Leadership Institute Class of 2021
- Operations Challenge 2020 to Begin Streaming Oct. 5
- Study: Citizen Science Critical to Sustainable Development
- Register for the First-Ever Remote Operations Challenge Competition
Comments are closed.
- Ultraviolet Expert Karl Linden Wins 2020 Clarke Prize January 22, 2021
- International Water Leaders Discuss Global Issues, Trends January 15, 2021
- Water Professionals Bring Rural Sanitation Solution to San Francisco December 10, 2020
Links of Interest
- Water Environment Federation
- Water Environment & Technology magazine
- Highlights archives
- January 2021
- December 2020
- November 2020
- October 2020
- September 2020
- August 2020
- February 2020
- January 2020
- December 2019
- November 2019
- October 2019
- September 2019
- August 2019
- February 2019
- January 2019
- December 2018
- November 2018
- October 2018
- September 2018
- August 2018
- February 2018
- January 2018
- December 2017
- November 2017
- October 2017
- September 2017
- August 2017
- February 2017
- January 2017
- December 2016
- November 2016
- October 2016
- September 2016
- August 2016
- February 2016
- January 2016
- December 2015
- November 2015
- October 2015
- September 2015
- August 2015
- February 2015
- January 2015
- December 2014
- November 2014
- October 2014
- September 2014
- August 2014
- February 2014
- January 2014
- December 2013
- November 2013
- October 2013
- September 2013
- August 2013
- February 2013
- January 2013
- December 2012
- November 2012
- October 2012
- September 2012
- August 2012
Advances in the integration of microalgal communities for biomonitoring of metal pollution in aquatic ecosystems of sub-Saharan Africa
- Review Article
- Open access
- Published: 01 June 2024
Cite this article
You have full access to this open access article
- Mary Mulenga ORCID: orcid.org/0009-0008-9198-280X 1 , 4 ,
- Concillia Monde ORCID: orcid.org/0000-0001-9856-9120 2 , 4 ,
- Todd Johnson ORCID: orcid.org/0000-0001-6346-5604 1 ,
- Kennedy O. Ouma ORCID: orcid.org/0000-0003-2902-9723 2 &
- Stephen Syampungani ORCID: orcid.org/0000-0003-2629-5807 3 , 4 , 5
133 Accesses
Explore all metrics
This review elucidated the recent advances in integrating microalgal communities in monitoring metal pollution in aquatic ecosystems of sub-Saharan Africa (SSA). It also highlighted the potential of incorporating microalgae as bioindicators in emerging technologies, identified research gaps, and suggested directions for further research in biomonitoring of metal pollution. Reputable online scholarly databases were used to identify research articles published between January 2000 and June 2023 for synthesis. Results indicated that microalgae were integrated either individually or combined with other bioindicators, mainly macroinvertebrates, macrophytes, and fish, alongside physicochemical monitoring. There was a significantly low level of integration (< 1%) of microalgae for biomonitoring aquatic metal pollution in SSA compared to other geographical regions. Microalgal communities were employed to assess compliance (76%), in diagnosis (38%), and as early-warning systems (38%) of aquatic ecological health status. About 14% of biomonitoring studies integrated microalgal eDNA, while other technologies, such as remote sensing, artificial intelligence, and biosensors, are yet to be significantly incorporated. Nevertheless, there is potential for the aforementioned emerging technologies for monitoring aquatic metal pollution in SSA. Future monitoring in the region should also consider the standardisation and synchronisation of integrative biomonitoring and embrace the “Citizen Science” concept at national and regional scales.
Graphical abstract
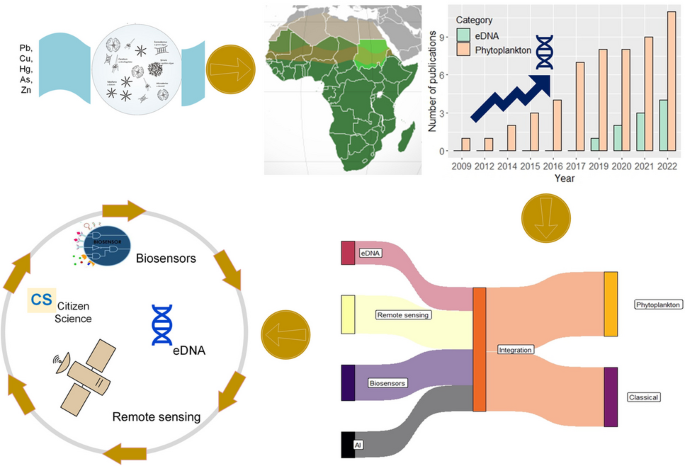
Avoid common mistakes on your manuscript.
Introduction
Stream ecosystems play an ecologically significant role in supporting aquatic biodiversity and providing beneficial ecosystem services that sustain the environment and promote human well-being (Limburg 2009 ; Maes et al. 2020 ). Stream ecosystem services include fresh water and food provisioning, sediment retention and transport, pollution control, recreation and ecotourism, flood regulation, disease prevention, nutrient cycling, and cultural heritage preservation (MEA 2005 ). In addition, both stream and riparian systems serve as biodiversity banks for aquatic and semi-aquatic biota as well as developmental stages of several terrestrial fauna such as arthropods, amphibians, and reptiles, among others (Mccabe 2010 ; Steward et al. 2022 ). Streams are also critical in transferring nutrients, matter, and energy, thus acting as sources and sinks of pollutants and disease vectors (Limburg et al. 2013 ; Wohl 2018 ; Bashir et al. 2020 ).
However, the negative impact of both natural and anthropogenic pressures has reduced the ability of stream ecosystems to supply aquatic ecosystem services (Khatri and Tyagi 2015 ). Natural factors such as climate change, droughts, floods, and other natural disasters impact aquatic ecosystems by altering water availability, water quality, and aquatic biodiversity (Costanza et al. 2014 ; Talbot et al. 2018 ; Culhane et al. 2019 ). Anthropogenic factors such as waste disposal, urbanisation, demand for agricultural land and expansion of industrial activities such as metal mining and fossil fuel combustion, and habitat destruction are also significant drivers of deterioration in aquatic ecosystems (Borgwardt et al. 2019 ; Cormier et al. 2019 ; Kimirei et al. 2021 ; Ferreira et al. 2023 ). Metal pollution of aquatic ecosystems from natural and anthropogenic sources is particularly an environmental and health concern in metal mining regions due to metals being persistent, non-biodegradable, and toxic (Yahya et al. 2018 ; Ali and Khan 2019 ; Amoatey and Baawain 2019 ; Zhou et al. 2020 ). In the stream ecosystems of Sub-Saharan Africa (SSA), aquatic metal pollution is an ever-growing environmental concern (Biney et al. 1994 ; Fayiga et al. 2018 ). There has been a steady accumulation of metals in water, sediment, and aquatic biota in rivers and lakes of SSA, mainly from natural and anthropogenic sources (Zhou et al. 2020 ; Yabe et al. 2010 ; Fayiga et al. 2018 ; Ochieng et al. 2009 ). In southern Africa, aquatic metal pollution above permissible limits has been reported for stream water and sediments from mining, coal use, and other industrial activities (Ouma et al. 2022 ; Addo-Bediako et al. 2021 ). Gerber et al. ( 2015 ) and Moyo et al. ( 2015 ) noted high Cu, Co, Pb, and Mn in the Olifants River associated with anthropogenic activities and posed a high risk to aquatic biota. Furthermore, Chetty and Pillay ( 2019 ) linked the influence of anthropogenic activities to elevated Cr, Cu, Pb, and Zn in Palmiet and Sezela rivers in South Africa’s Kwa-Zulu Natal coupled with high mobility and bioavailability. In the Zambian Copperbelt, Cu, Co, Pb, and Zn mining has impacted the water and sediments of Lake Kariba (Chalumba et al. 2021 ) and Kafue River with increased ecological risks to aquatic life. In the Katangese Copperbelt of the Democratic Republic of Congo, extreme sediment enrichment with Cu (190.2 mg/kg) and Zn (1117 mg/kg) in the Bumbu River draining Kinsasha has been reported (Kayembe et al. 2018 ). Banze wa Mutombo ( 2022 ) also associated the high pollution of the Mura and Kimpulande tributaries of the Congo River with Cu-Co-As-Cd-loaded mining effluents that increased the vulnerability of aquatic communities.
Metal pollution in West Africa’s aquatic systems has similarly reached alarming levels. Gbogbo and Otoo ( 2015 ) reported the detrimental impacts of Cd, As, Hg, and Cu pollution on water, macrophytes, algae, and fish in Ghana’s Sakumo II wetland in the Tema Metropolitan area. According to Ngueyep et al. ( 2021 ), Cameroon’s Kadey River tributaries had excess Ni, Fe, Cr, Se, As, and Hg in sediments from artisanal and small-scale gold mining. Tyovenda et al. ( 2019 ) reported contamination of water and algae and sediment enrichment with Pb, Hg, Ni, and Fe in River Benue, Nigeria. Despite the relatively low mineral deposit in Eastern Africa, alarming aquatic metal pollution has been reported in Kenya’s gold mining belt (Ngure et al. 2014 ), Tanzania’s Mara River (Nkinda et al. 2020 ), Awetu watershed in Ethiopia (Astatkie et al. 2021 ), and Namukombe stream in Uganda (Omara et al. 2019 ). For instance, Ngure et al. ( 2014 ) noted high Hg (355 mg/kg) in fish, while Astatkie et al. ( 2021 ) recorded stream sediment contamination with Pb (2,000 mg/kg), As (623 mg/kg), and Cr (375 mg/kg). Based on the representative studies above, there is sufficient evidence of aquatic metal pollution thus, raising the need for monitoring of aquatic ecosystems across SSA.
One of the approaches that can be employed to effectively monitor and assess the magnitude of anthropogenic and natural impacts on stream ecosystems is the bioindicator concept, which utilises sentinel aquatic biota (Lazorchak et al. 2003 ; Schwacke et al. 2013 ; Parmar et al. 2016 ). Bioindicators reflect the bioavailable fractions of pollutants and hence are of potential ecotoxicological significance (Hamza-Chaffai 2014 ; Lamare 2019 ; Kumari and Paul 2020 ) Based on the targeted outcome, three categories of bioindicators commonly used for monitoring environmental health include early warning, compliance, and diagnostic indicators (Hamza-Chaffai 2014 ). “Early-warning bioindicators” signify the impending deterioration of ecosystem health. Deviations from the acceptable aquatic environmental conditions are detected by “compliance indicators” while the “diagnostic bioindicators” reflect the causes for the deviations from the expected ecological conditions of the aquatic ecosystem (Sumudumali and Jayawardana 2021 ). Figure 1 illustrates the bioindicator concept of aquatic metal pollution biomonitoring that utilises the compliance, diagnostic, or early-warning aspects of indicator species or communities.

Conceptualising integrative monitoring of aquatic metal pollution in stream ecosystems
Aquatic microalgal communities have been utilised to monitor the ecosystem health in lotic environments (Yilmaz et al. 2021 ; Feisal et al. 2023 ). The vital ecological role of microalgal communities has been recognised through continuous surveillance to establish their status in the aquatic environment (Li et al. 2021 ; Thompson and Carstensen 2023 ). The ability of microalgae to accumulate high levels of pollutants, their relative sessile nature, ease of sampling, ease of culture in the lab, and their trophic importance as primary producers in the aquatic food web have positioned them as suitable bioindicators of metal pollution (Zhou et al. 2008 ; Parmar et al. 2016 ; Kumari and Paul 2020 ). Freshwater microalgae occur either as planktonic, which dominate the pelagic zone, or the benthic forms associated with substrates such as sediments, rocks, macrophytes, mud, and organic debris (Bellinger and Sigee 2015 ).
Globally, the use of microalgae for biomonitoring as early-warning signals has been widely documented. For instance, in Europe, Dokulil et al. ( 2016 ) documented long-term historical biomonitoring using microalgae responses and community composition in the extensive Danube River hydro system. Furthermore, biomonitoring of metal pollution in the transboundary Danube River delta aquatic complex reported high concentrations of bioavailable Ni, Cd, and other potentially toxic elements (Burada et al. 2015 ; Simionov et al. 2021 ; Calmuc et al. 2021 ). Metal pollution trends were also observed in the southeastern Brazil river basin impacted by metal contamination from the Mariana dam failure, with increased Hg bio uptake by microalgae (Marques et al. 2022 ). Silva et al. ( 2022 ) further reiterated the significance of using morphological and taxonomic responses of microalgae as bioindicators to environmental changes in river basins of southern Brazil. In India, microalgal communities in the tropical freshwater Godavari River, Cu, and Zn exhibited lethal effects at high concentrations for the dominant cyanobacteria and chlorophytes (Chakraborty et al. 2010 ). Feng et al. ( 2021 ) also noted the detrimental impacts of metal pollution on the microalgal community structure, with certain microalgal species being more sensitive to the bioavailable metals in the Yangtze River in China. In the Sefid Rud River, Iran, changes in microalgal assemblages were suitable bioindicators of environmental variability and corresponded to physical and chemical changes in the south Caspian Sea catchment (Ramezanpour et al. 2014 ).
Microalgal communities have also been used to monitor metal pollution in Africa’s stream ecosystems. In West Africa’s Niger River system, Ezewudo et al. ( 2021 ) noted weak to high potential ecological risks to aquatic communities, including microalgae, from As, Cd and Hg contamination. In the Cameroon Centre Region, the spatial-seasonal changes in algal densities in the streams of the Sanaga lotic system network draining urban and industrial settlements corresponded to changes in the aquatic physicochemical environment (Pascale 2023 ). According to Mangadze et al. ( 2019a ), several ecological health studies on southern Africa’s rivers have applied benthic diatoms for biomonitoring. Dalu et al. ( 2014 ) noted a direct response of microalgal communities to changes in the physicochemical environment of the Kowie system riverine-estuarine continuum in South Africa’s Eastern Cape. Recent studies on South Africa’s urban Molopo River depicted anthropogenic Cu, Cr, Zn, and Pb sediment contamination with potentially deleterious ecological impacts on the benthic algal and macrofauna communities (Mohajane and Manjoro 2022 ). Additionally, the diatom-based biomonitoring tools (e.g., the “South African Diatom Index (SADI)” and the “Benthic Diatom Index (BDI)”) have been used to detect and quantify the magnitude of natural and anthropogenic impacts on stream ecosystems (Lang et al. 2013 ; Harding and Taylor 2014 ; Sirunda et al. 2021 ).
Microalgae have the potential to be integrated into conventional monitoring programs as complementary tools to increase the resolution in detecting sub-lethal contamination and thus serve as early-warning bio-systems (Cid et al. 2012 ; Bae and Park 2014 ). Despite the potential of integrating microalgal communities in the biomonitoring of aquatic ecosystems, this approach remains one of the least explored alternatives to sustainable management of freshwater ecosystems in SSA (Lemley et al. 2016 ). Therefore, this review seeks to (1) provide insights into recent advances in the integration of microalgae in biomonitoring metal pollution in the SSA lotic systems, (2) highlight the potential of integrating microalgal as bioindicators in the emerging technologies for monitoring aquatic metal pollution of lotic systems, and (3) identify research gaps and suggest directions for further research in the integrating microalgae as bioindicators of metal pollution in lotic systems of SSA.
Methodological approach
Scope of literature search.
To ensure that high-quality and relevant articles were selected, our review defined explicit inclusion criteria outlined in Cornelissen et al. ( 2009 ). The literature search included articles addressing advances in integrating microalgae for biomonitoring metal pollution in stream ecosystems draining metal-mining landscapes of SSA. The search was restricted to original research, written in English, from articles published between January 2000 and June 2023 to identify ‘gold-standard’ literature on stream biomonitoring of metal pollution with a focus on microalgae as bioindicators.
The article selection process aims to identify the original research papers that present clear evidence of the study objectives (Syeed et al. 2023 ). Page et al. ( 2021 ) preferred reporting items for systematic review and meta-analysis (PRISMA) protocol was followed to ensure a comprehensive and well-defined strategy for the identification, screening, and inclusion of articles for review (Fig. 2 ). Reputable academic databases, SCOPUS, Taylor and Francis, and Semantic Scholar were searched for authentic articles (Kitchenham and Charters 2007 ). Furthermore, snowballing or citation-searching from “gold-standard” literature was used to identify more articles for preliminary screening (Wright et al. 2014 ).
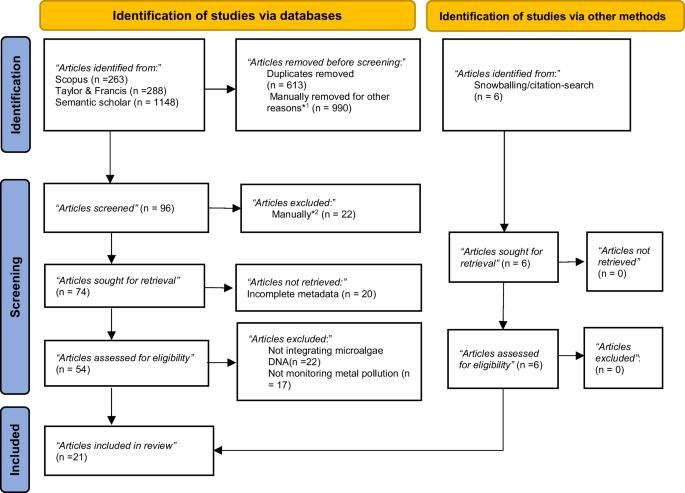
The PRISMA protocol for identifying, screening, and including literature for the review
The literature search was conducted based on the article title, abstract, and keywords using key terms such as “bioindicator”, “aquatic biomonitoring”, “metal mining”, “aquatic pollution”, “algae”, “aquatic ecosystems”, “sub-Saharan Africa”, “Africa”, “e-DNA”, “environmental DNA”, “metagenomics”, “microalgae”, “Biosensors”, “ Remote sensing ”, and “ Citizen science ”. From each article included, the following information was extracted: (i) title, (ii) authors, (iii) publication year, (iv) regional distribution (v) main objective, (vi) methods (physical, chemical, biological) (vii) indicator organism(s), (viii) environment (sediment, water, biota), (ix) pollutant(s) (x) microalgal metrics used, and (xi) key findings, gaps, or recommendations.
Streamlining article evaluation and selection
Quality assurance and bias reduction.
To remove bias in the first stage of the search, the authors searched independently in the digital databases using search terms with slightly varying synonyms to maximise the extraction of articles from the global search. This initial search was followed by the within-results search, where the authors used the same filter criteria specifying the period, the document type, the region of study, and the field of study. In the second stage, the authors verified the extracted articles’ metadata for completeness and originality. Articles that fulfilled the quality assurance process were included for further synthesis.
Article processing
The results from the databases were downloaded and imported into the Mendeley reference software (Mendeley Ltd). The following metadata was checked and updated where necessary for each article: author(s), title, year of publication (and month), volume, page numbers or article number, abstract, keywords, and DOI, if available. However, articles for which pertinent metadata items such as author, title, or publication year that were missing were further excluded from the list.
Exclusion process
An automated keyword-based search was used to explore the database and extract relevant research articles (Beecham et al. 2008 ). The terms were searched in the article titles, abstracts, and keywords. The exclusion criteria for out-of-scope articles were principally based on the following aspects: (1) studies outside freshwater systems, e.g., oceans and seas; (2) other bioindicator categories used, e.g., non-photosynthetic bacteria, marine plankton, freshwater zooplankton, macrophytes, macroalgae, macroinvertebrates, and vertebrates; (3) clinical and laboratory biomonitoring studies, e.g. humans and wild and domestic animals using water resources; and (4) studies involving aquatic ecosystem pollutants other than metals. Furthermore, manual removal was conducted to ensure that only relevant and complete articles were included in the final review process (Petticrew and Roberts 2008 ).
Bibliometric analysis
A bibliometric analysis of the extracted information was conducted to classify articles based on the year of publication, authors, region, main objective(s), bioindicator type, environmental matrix, pollutant(s), methods, and the microalgal metric(s). Following the PRISMA filtering protocol, the review included 21 articles (15 from the digital scholarly databases and six from snowballing/citation search) relevant to the research area, geographical location, and study period. From Fig. 3 a, between January 2000 and June 2023, there was a notable general cumulative 95% increase in the studies incorporating different microalgal taxa in monitoring metal pollution in aquatic ecosystems in the SSA. This indicates a growing interest in incorporating microalgal taxa in aquatic biomonitoring.
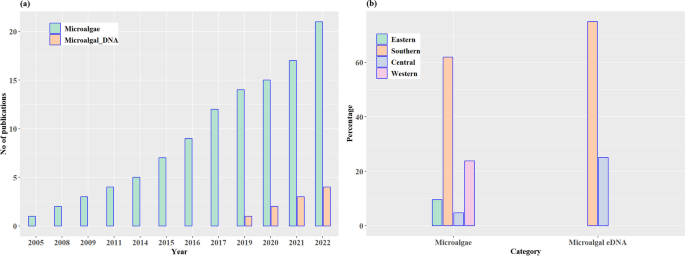
a Distribution of publications by year and research focus and b regional proportions of microalgae and environmental DNA (eDNA) integration in the monitoring of metal pollution in aquatic ecosystems of sub-Saharan Africa
Generally, between January 2000 and June 2023, studies indicate that only South Africa, Namibia, and the Democratic Republic of Congo (DRC) integrated microalgae and microalgal-based eDNA, respectively, in aquatic biomonitoring for metal pollution. Sub-regionally, only 24% of the countries in West Africa, 10% in Eastern Africa, 25% in Central Africa, and 75% in Southern Africa conducted microalgal-based biomonitoring of metal pollution in streams. However, microalgal-eDNA integration in aquatic metal pollution biomonitoring is still in its infancy in SSA, with only Central and Southern Africa accounting for 25% and 75% of aquatic-based research to monitor metal pollution (Fig. 3 b). The integration of microalgal-eDNA method in aquatic metal pollution biomonitoring in SSA was first documented by Jordaan et al. ( 2019 ), who noted a 6% variability in bacterial community composition and diversity from the anthropogenic Co, As, Cr, Ni, and U pollution in the rivers within the lower Wonderfonteinspruit catchment of South Africa. Since then, the eDNA approach has been seen as a potential approach to accelerate aquatic biomonitoring by supplementing traditional taxomorphological monitoring in the SSA landscape (Perry et al. 2022 ).
Bioindicator taxa and environmental assessment
The diatoms are the single most preferred microalgal bioindicator taxon (36%) and are also used with benthic macroinvertebrates (18%) to monitor aquatic metal pollution. Considering their specificity and sensitivity to ecological changes in aquatic ecosystems, diatoms have been widely employed to detect perturbations in stream water quality (Lobo et al. 2016 ; Mangadze et al. 2017 ). The preference for both taxa could be attributed to their stationary and benthic nature, which makes them suitable for recording long-term pollutant trends compared to the instantaneous physicochemical methods that only consider a “snap-shot” of the environmental water quality (Beyene et al. 2009 ; Hattikudur et al. 2014 ). Other single-use taxa of microalgae, including cyanobacteria, had equal preferences (18%), while “algae” and macroinvertebrates comprised 9% each as the bioindicators used to assess metal pollution (Fig. 4 a).
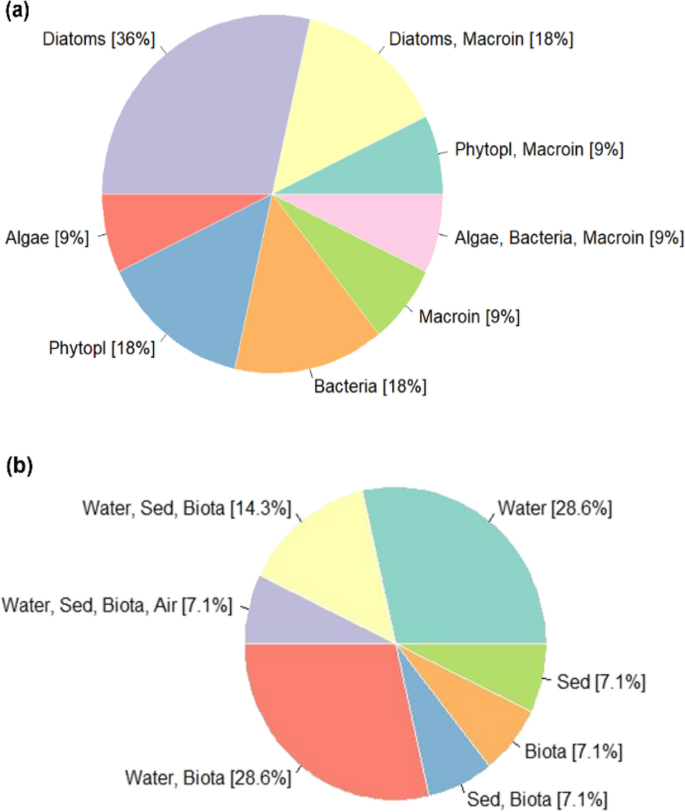
a Bioindicator integration in aquatic metal pollution biomonitoring in SSA; b environmental matrices investigated for metal pollution. Macroin macroinvertebrates, Phytopl phytoplankton, Sed sediment
Regional studies elsewhere, for example, in North America (e.g., Smucker et al. 2018 ) and Asia (e.g., Chon et al. 2013 ), have similarly integrated algae and macroinvertebrates as well as microbial communities to monitor metal pollution of freshwaters while leveraging on the producer–consumer trophic changes as indicators of disturbances at catchment scale. Furthermore, Respondek et al. ( 2022 ) integrated mosses and microalgae in monitoring metal pollution in surface water in the smelter area of Ozimek, Poland. They observed diatom taxa as the dominant algal group that indicated responses to metal stress, e.g., the metal-tolerant Achnanthidium sp and Mayamaea sp dominated up to 99% of the algal communities, and served as excellent bioindicators of metal contamination. In addition, Pandey ( 2020 ) compared green algae , cyanobacteria, and diatom species and noted an increased relative taxa abundance, indicating increased tolerance to metal pollution. Moreover, increased lipid production and cell-wall teratologies in diatoms, also indicated by Lavoie et al. ( 2012 ), were observed under Cu, Cd, Zn, and Pb stress. Pandey and Bergey ( 2018 ) also found that diatoms-dominated periphyton biofilms were excellent indicators of metal pollution, thus showing the utility of periphytic diatom communities as an effective tool for biomonitoring of aquatic metal pollution. Gbogbo and Otoo ( 2015 ) used the biomonitoring potential of algae, among other bioindicator biota of an urban wetland system in Ghana, to determine the magnitude of metal pollution algae accumulated up to 12 mg/g Cd. Similarly, Leguay et al. ( 2016 ) and Solak et al. ( 2020 ) reiterated the importance of complementing physicochemical assessment techniques with diatom-dominated biofilm-based proxies, diatom indices (e.g., the Pampean Diatom Index and Specific Pollution Index) to monitor metal contamination in aquatic systems.
In Fig. 4 b, water is the most frequently assessed abiotic matrix (28.6%) and in combination with different bioindicators in the same proportion for assessing metal contamination. Studies by Dalu et al. ( 2017 , 2022a ) and Tyovenda et al. ( 2019 ) included stream sediment plus water, diatoms, algae, and benthic macroinvertebrates in the evaluation of metal pollution to obtain a three-way health status of the aquatic ecosystem. In addition, Mangadze et al. ( 2017 ) incorporated a fourth dimension of atmospheric contribution to stream ecosystem metal pollution to assess the potential of using diatoms as suitable bioindicators of ionic metal pollution along a South African temperate river system.
Application of microalgae for biomonitoring tropical stream ecosystems of SSA
In Fig. 5 and Table 1 , three studies (by Jordaan et al. 2019 Laffite et al. 2020 Perry et al. 2022 ) incorporated the microalgal-eDNA to check for environmental compliance with the established national or international guidelines for metal contaminant levels in freshwater aquatic environments. Jordaan et al. ( 2019 ) and Pereira‐da‐Conceicoa et al. ( 2021 ) used eDNA as a diagnostic tool to determine the causes of deteriorating water quality and changes in microbial communities in South African river catchments. However, no study used microalgal-eDNA for early warning of aquatic ecosystem change, making this a potential area for future research. Most studies (76%) employed various microalgal taxa responses for compliance monitoring, followed by diagnostic and early-warning functions, each at 38% (Fig. 5 ).
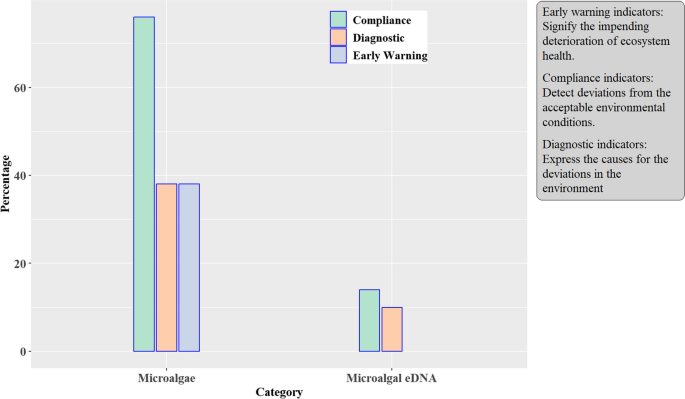
Application of the microalgal taxa in the integrated monitoring of the environmental conditions of aquatic ecosystems exposed to metal pollution in SSA
Several studies combined more than one environmental application of algal communities to test for compliance, diagnosis, or early warning to evaluate the overall integrity and potential ecological risks for the respective aquatic ecosystems investigated. Despite its environmental importance, only 30% of the studies used biomonitoring as an early-warning tool, while 40% employed biomonitoring for diagnostic purposes. Nevertheless, 91% of the studies were targeted to determine environmental compliance of anthropogenic activities that introduce metal contaminants to the aquatic environment against the set effluent discharge standards in the pro-active management of aquatic ecosystems in SSA. Table 1 highlights the environmental applications (compliance, diagnostic, and early warning) of microalgal communities based on the main objectives of the reviewed studies. Algal communities are helpful for compliance, diagnostic, or early-warning biomonitoring since they reflect long-term changes in stream water quality (Mangadze et al. 2016 ) (Table 1 ). Ugbeyide and Ugwumba ( 2021 ) assessed the physicochemical and biological status of the Ibuya River in Nigeria, which was impacted by anthropogenic pollution. Cd (0.003 mg/L) and Pb (3.5 mg/L) levels exceeded permissible limits for surface water quality, while the lower species richness and composition, dominated by Bacillariophyceae, reflected a lotic system impacted by allochthonous pollution. Oberholster et al. ( 2016 ) observed increased algal species diversity caused by improved downstream water quality during the rehabilitation of the Grootspruit wetland, South Africa, impacted by acid-mine drainage. The trends concur with a previous study by Ali and Abd el-Salam ( 1999 ) that noted changes in the dominance of microalgal species Cyclotella and Nitzschia (Bacillariophyta), Actinastrum and Scenedesmus (Chlorophyta), and Oscillatoria sp (Cyanophyta).
Furthermore, in the Macedonian Maidanska River, the bioconcentration and biomineralisation of Cu, As, Cr, Se, and Cs were observed in Audouinella sp, while the high bioaccumulation of Ba (3 mg/g) and intracellular biomineralisation were evidenced in Spirogyra sp. thereby positioning these algal species as a biological pathfinder for acid-mine drainage deposits (Bermanec et al. 2018 ). Water and sediment chemistry, including nutrient and metal pollutants, largely influence the stream algal community composition. Dalu et al. ( 2017 ) explored the influence of anthropogenic impacts on diatom communities and noted the dominance of pollution-tolerant taxa in an austral temperate stream in South Africa. The tolerance and morphological changes (teratologies) on epilithic diatom communities have also been employed to monitor and quantify the biological effects of metal stress from an abandoned Coval da Mo mine drainage (Ferreira da Silva et al. 2009 ). The findings agree with Pandey and Bergey ( 2018 ), who correlated non-taxonomical parameters, including teratologies and lipid bodies, to indicate metal toxicity and recovery in fluvial systems. Diatom indices, including the GDI (Generic Diatom Index), BDI (Biological Diatom Index), and TDI (Trophic Diatom Index), were successfully employed to monitor the Dongjiang River in China with BDI and GDI showing an apparent response to water quality changes (Deng et al. 2012 ). Diatoms have also been incorporated in multispecies biomonitoring of the temporal variability of metal pollution in Nigeria’s Calabar River (Hena et al. 2022 ) and Kebena-Akaki Rivers, Ethiopia (Beyene et al. 2009 ). In both studies, a significant response was observed between the algal community structure and metal concentrations. Mangadze et al. ( 2017 ) similarly reflected the role of diatom assemblages as bioindicators of metal pollutants (e.g., As, Zn, Cu, and Cr), particularly on low pollution tolerant species such as Fragilaria , Cyclostephanos , and Gyrosigma transition to high pollution tolerant forms (e.g., Nitzschia and Gomphonema ). This observation is also supported by findings in Dalu et al. ( 2022b ), where changes strongly influenced the structure of diatom communities in water and sediment quality due to the presence of metal contaminants such as B, Cu, and Fe in the Krom River system of the western cape, South Africa. Microalgal communities have also been used to indicate metal pollution in lacustrine systems. For instance, Ogoyi et al ( 2011 ) determined metal concentrations (Zn, Pb, Cd, Cr, and Hg) in algal communities alongside water and sediment as an integrative aquatic ecosystem assessment approach.
Integrating microalgal communities into molecular tools for compliance, diagnostic, or early-warning monitoring of streams in mining regions of SSA is also ongoing. Jordaan et al. ( 2019 ) studied the influence of anthropogenic pollution on the structure and function of aquatic bacterial communities, using 16S rRNA as a proxy indicator, in South Africa’s Wonderfonteinspruit river catchment. Pereira-da-Conceicoa et al. ( 2021 ) demonstrated the merits of incorporating eDNA into existing aquatic biomonitoring metrics with the potential of recovering more diversity and a higher resolution. The ecological advantages of integrating eDNA studies in aquatic biomonitoring above are also evident in other global investigations. Li et al. ( 2018 ) noted that the operational taxonomic units of molecular e-DNA data can predict up to 79% of aquatic pollution. Ancion et al. ( 2010 ) used 16S rRNA gene libraries to examine the impact of Cu, Zn, and Pb on bacterial communities embedded in freshwater biofilms and recorded higher sensitivities, thereby confirming their potential role as compliance indicators of stream health.
Method integration and environmental and biological metrics used for assessment of metal pollution in aquatic ecosystems of SSA
All the reviewed microalgal-based works combined physicochemical and biological techniques to investigate metal pollution, possibly to enhance the detection of contaminants and their impact on biota (Torrisi et al. 2010 ). Several environmental and biological indices were used to quantify the magnitude of the impact of metal pollution, including enrichment and contamination factors, pollution indices, species richness, and diversity indices (Bere et al. 2016 ; Dalu et al. 2022a ; Mangadze et al. 2019b ; Ugbeyide and Ugwumba 2021 ) (Table 2 ). According to Lobo et al. ( 2016 ), biotic indices such as Beck’s index and Renberg’s “Index B” developed from the relative abundances of bioindicator species have been employed for biomonitoring of streams and other aquatic ecosystems. The determination of the physicochemical water quality coupled with the estimation of aquatic biodiversity based on biotic indices has been used to infer the ecological health status and as “early-warning” indicators of aquatic ecosystem health changes (Bellinger and Sigee 2015 ; Forio and Goethals 2020 ). Geochemical indices such as contamination factor (CF), enrichment factor (EF), geo-accumulation index (I geo ), and pollution load index (PLI) were used to evaluate the occurrence and magnitude of pollution in SSA streams receiving metal(loid) contaminants (Tyovenda et al. 2019 ; Hena et al. 2022 ). Changes in algal community composition, abundance, PLI, and metal pollution index (MPI) have also been used to assess aquatic metal pollution stress in aquatic communities of Egypt’s Alexandria coast (Ismail and El Zokm 2023 ).
Integrating molecular techniques in biomonitoring is a potential approach to revolutionise aquatic pollution assessment (Li et al. 2010 ; Lobo et al. 2016 ). In this review, Laffite et al. ( 2020 ) observed a significant correlation between metals and 16 s rRNA, suggesting a close link between metal pollution and human-mediated pressures on an urban river in the Democratic Republic of Congo. Pereira-da-Conceicoa et al. ( 2021 ) demonstrated the relevance of integrating environmental DNA (eDNA) into existing monitoring metrics to provide additional taxonomic resolution for aquatic biodiversity management in South African streams. The application of molecular methods has also been observed to substantially improve the biomonitoring of streams in France, China, and Switzerland compared to the traditional morphotaxonomic methods (Apothéloz-Perret-Gentil et al. 2021 ; Keck et al. 2018 ; Li et al. 2018 ). However, Perry et al. ( 2022 ) noted a significant drawback in the integration of eDNA principally inhibited by inadequate reference data for SSA in the gene banks. The lack of reference eDNA databases, downstream transport, dilution of DNA fragments, and introduction of terrestrial DNA, among other challenges, has also been observed in other regions, e.g., Finland (Norros et al. 2022 ), Switzerland (Deiner et al. 2016 ), Canada (Laporte et al. 2022 ), and globally (Beng and Corlett 2020 ).
Integrating microalgae into emerging technologies for monitoring metal pollution in stream ecosystems
Several cutting-edge emerging technologies are gaining popularity as complementary approaches to support conventional monitoring and assessments of stream ecosystems, as described below.
Microalgal-eDNA metabarcoding
Based on the current review, recently, limited studies have incorporated microalgal eDNA in biomonitoring aquatic metal pollution in the SSA. For instance, Laffite et al. ( 2020 ) investigated the co-contamination and seasonal variability of metal in bed sediments of urban rivers in DRC using bacterial eDNA. Significant correlations were observed between metal concentrations and 165 s rRNA bacterial densities, linking pollution to anthropogenic inputs. In South Africa, Jordaan et al. ( 2019 ), using the 16 s rRNA gene profiles, noted a substantial impact of pH and metal contamination from mining on bacterial diversity and community structure in the lower Wonderfonteinspruit catchment rivers. Furthermore, Perry et al. ( 2022 ) demonstrated the cost–benefit of using bulk samples and eDNA for multispecies biodiversity monitoring of Namibia’s freshwater systems. However, in most SSA countries, few studies, if any, have integrated microalgal eDNA in aquatic metal pollution biomonitoring. Given the sparsity of eDNA biomonitoring research data in SSA, more effort is needed to develop methods adapted to regional and local conditions and to generate eDNA gene-bank reference data to increase our understanding of SSA aquatic ecosystems (Perry et al. 2022 ). In addition, the performance of eDNA tools in biomonitoring aquatic metal pollution in SSA lotic ecosystems compared with the conventional monitoring approaches is not adequately investigated. Therefore, further research is needed to address this methodological gap by integrating microalgae-based eDNA biomonitoring of aquatic metal(loid) pollution at the community, species, and molecular level in stream ecosystems of SSA (Stat et al. 2017 ).
In the recent past, most of the eDNA biomonitoring has been conducted in the global North (Resh 2007 ). For instance, Cilleros et al. ( 2019 ) compared the effectiveness of eDNA metabarcoding and conventional morphotaxonomic techniques while assessing the diversity of fish assemblages in 38 streams of the French Guiana. Their findings revealed that while traditional taxonomic methods offered a more comprehensive inventory of fish taxa, they were spatially limited. In contrast, eDNA metabarcoding, when complemented with classical methods, was a more comprehensive and efficient approach for rapidly assessing and monitoring fish diversity on a larger spatial scale. Similarly, Gleason et al. ( 2021 ) conducted a study in southern Ontario, Canada, comparing eDNA metabarcoding techniques with traditional kick-net sampling to monitor lotic macroinvertebrate communities. Their findings demonstrated that eDNA techniques, especially metabarcoding of bulk tissues, provided a better representation of the diversity of macroinvertebrate taxa at a finer spatial resolution than traditional methods. However, in SSA, few studies have integrated eDNA in aquatic biomonitoring of metal pollution (e.g., Laffite et al. 2020 ; Jordaan et al. 2019 ; Perry et al. 2022 ). Therefore, progressive regional research must be strengthened to overcome the current limitations of aquatic eDNA biomonitoring, such as inadequate e-DNA reference data (Perry et al. 2022 ).
While acknowledging that eDNA is more appropriate for short-term monitoring, eDNA data can be used integratively with long-term monitoring approaches, such as remote-sensing, biosensor, and citizen science (Hansen et al. 2020 ). For instance, eDNA data can be used to validate or ground-truth remotely sensed data to ensure the reliability of long-term monitoring systems. Additionally, the integration of eDNA can increase the resolution of pollutant detection at sub-lethal and ensure the validity and consistency of sensed data.
Biosensor systems for aquatic biomonitoring
Recently, a variety of biosensors gained high attention and have been employed in in-situ for real-time monitoring and detection of environmental contaminants (Huang et al. 2023 ). A biosensor typically comprises a biosensing probe and a transducer that detects a contaminant by producing a quantifiable signal (Mishra et al. 2019 ; Rovira and Domingo 2019 ). The biosensor probe material can be antibody-, DNA-, whole-cell-, or enzyme-based (Singh et al. 2020 ). The transducer translates the biological signals to optical or electrical signals via optical, physicochemical, or piezoelectric material (Nguyen et al. 2019 ). Electrochemical and optical biosensors have been employed to detect and quantify metals, including Hg + , Pb 2+ , Zn 2+ , Cu 2+ , and Cd 2+ in water (Wu et al. 2023 ). Advances in nanotechnology have further improved the performance of biosensors due to the numerous benefits of larger sensing equipment. Nanosensor materials improve biosensor efficiency for colour sensing, target sensitivity, and carrier capacity. Additionally, nanomaterials have high thermal and electrical conductivity (Huang et al. 2021 ; Abdel-Karim 2024 ).
Whole-cell microbial biosensors detect metal ions based on the genetic element that responds to target metals (Huang et al. 2023 ). In aquatic environments, whole-cell bacterial biosensors have been used to detect bioavailable metals with high sensitivity (Cerminati et al. 2015 ). Alfadaly et al. ( 2021 ) applied a complementary target resistive Rhizobium bacteria-based and Rhodotorula fungi-based bioelectrochemical sensor to detect and remove Cr 6+ and Cd 2+ ions from polluted water. The bacterial component exhibited superior performance for metal resistivity and removal. In another study, Cerminati et al. ( 2015 ) confirmed the efficacy of a broad-spectrum whole-cell-based metal biosensor as a screening tool for the presence of bioavailable Au, Hg, Pb, and Cd in water.
Genetically engineered DNA-based microbial biosensors combined with electrochemical transducers broaden the applicability of cell-based biosensors for early monitoring and detection of metal ions in water Jeon et al. ( 2022 ). According to Jeon et al. ( 2022 ), the mutation of a regulatory protein ZntR in Escherichia coli enhanced the selectivity of Pb 2+ ions after metal ion-exporting genes were deleted in the host cells. Furthermore, Nourmohammadi et al. ( 2020 ) observed high specificity for Pb 2+ bacterial biosensor expressing a luciferase reporter gene controlled by pbr / cadA promoters in Cupriavidus metallidurans in a genetically engineered bacterial system.
According to Huang et al. ( 2023 ), biosensors are low-cost, easy to use, and energy-saving and require minimal pre-sample treatment. In addition, biosensor technology uses non-hazardous materials and has considerably low carbon footprints compared to physicochemical methods. Furthermore, the integration of bacteria into biosensor technologies offers numerous benefits in the detection and monitoring of aquatic metal pollution. Biosensors and microalgae serve as complementary tools, offering different perspectives and capabilities. Biosensors enhance the detection and quantification of metal contaminants in real-time or near real-time, thereby allowing for rapid detection and tracking of metal pollution (Wu et al. 2023 ).
In contrast, microalgae are reliable bioindicators of long-term exposure to metal pollution, reflecting the historical trend. A combination of biosensor data with microalgae assessments reflects a comprehensive understanding of short and long-term metal pollution dynamics in impacted streams. Additionally, biosensors often exhibit high sensitivity and specificity for detecting target metal ions, enabling the detection of sub-lethal metal concentrations (Huang et al. 2023 ). Microalgae, while sensitive to metal pollution, may not always provide precise measurements of metal concentrations at low levels or in complex environmental matrices. The integration of microalgal DNA into biosensors has the advantage of sensitivity and specificity, especially in natural environments with multi-elemental metal contaminants. Furthermore, biosensors are robust and can simultaneously be deployed at multiple locations within stream ecosystems. This spatial advantage complements the localised application of microalgae per time to monitor metal contamination.
Additionally, biosensors are often portable and easy to deploy, making them accessible for field-based monitoring in remote or challenging environments. However, the high cost and technological requirements of nanomaterials production could impede the production and application of nanobiosensors, particularly in developing countries.
Remote sensing
Satellite-based remote sensing (RS) and hyperspectral imaging is a cost-effective monitoring approach that enhances extensive and rapid spatial coverage of the Earth’s surface with repeatability capabilities for investigating environmental systems (Reddy 2018 ; Pettorelli et al. 2018 ). By detecting unique spectral signatures of various substances, including metals, RS can pinpoint the presence and concentration of specific metals in waters via hyperspectral electromagnetic radiation. For instance, Lin et al. ( 2024 ) determined the concentration of metals in China’s Dalian Lake using hyperspectral analysis and genetics algorithms. The integration of RS techniques with biosensor data capture probes also improved the spatial mapping of metals and sediments along Egypt’s Red Sea Coast (Mohammed et al. 2024 ). Bresciani et al. ( 2016 ) mapped patterns of cyanobacterial blooms in five Italian lakes using a suite of aerial and space-borne hyperspectral sensors with increased accuracy. Guo et al. ( 2022 ) and Cao et al. ( 2018 ) integrated RS to model metals and chlorophyll- a concentrations in water. The models provided high retrieval accuracy and realistic information.
Data generated from RS enables the creation of detailed spatiotemporal maps of aquatic metal pollution and hotspots mapping. Integrating RS data with water quality measurements and GIS data provides a comprehensive understanding of metal pollution dynamics in aquatic ecosystems (Yu et al. 2020 ; Zhu et al. 2022 ). Furthermore, RS is cost-effective and efficient compared to traditional field-based methods, enabling the rapid collection of extensive data over large areas with lower monitoring costs (Avtar et al. 2020 ). Overall, RS serves as a valuable tool in monitoring metal pollution in aquatic environments, providing timely and spatially explicit information crucial for informed decision-making and effective environmental management strategies.
The complementarity between RS and microalgae in monitoring metal pollution in aquatic systems is multifaceted. RS provides wide spatial coverage, allowing for the monitoring of large water bodies and the identification of metal pollution hotspots (Chi et al. 2016 ). However, RS may lack the spatial resolution needed to detect localised pollution events or variations. Microalgae, on the other hand, can be highly sensitive to rapid changes in metal concentrations and hence reflect localised pollution impacts. Furthermore, RS allows for the monitoring of changes in metal pollution over time by capturing images at different intervals. In contrast, the rapid response of microalgae to changes in metal concentrations makes useful indicators of short-term pollution events.
RS data can be validated and calibrated using ground-truthed data, including responses from bioindicators, including microalgae (Cook et al. 2023 ). This process enables researchers can assess the accuracy of remote-sensing-derived metal pollution estimates and refine remote-sensing algorithms to improve their reliability. Microalgae responses to metal pollution can serve as early warning indicators of environmental degradation. With a combination of RS data with real-time monitoring of microalgae populations, researchers can develop early warning systems to alert authorities to potential pollution events or ecosystem stressors, enabling timely intervention and mitigation efforts.
- Citizen science
Citizen science monitoring involves volunteers (i.e., mainly non-professionals), often the riparian communities, and is fundamentally public participation by stakeholders in environmental stewardship (Moharana 2021 ; Fraisl et al. 2022 ). Citizen science has the potential of upscaling field studies to a regional or global extent coupled with centralised monitoring efforts that enhance extensive and well-coordinated environmental monitoring, which can produce large datasets rapidly. Miguel-Chinchilla et al. ( 2019 ) analysed citizen-sensed catchment data on stream turbidity which contributed nearly 12% value to the study. Babiso et al. ( 2023 ) analysed water quality data collected from the Meki River, Ethiopia, by citizen scientists. The study results indicated a good agreement with selected parameters, which implied the accuracy of citizen-collected data. Additionally, Thornhill et al. ( 2017 ) used citizen science stream data from the metropolis of China to model and classify predictors of water quality using random forest models with reliable results.
The incorporation of smartphone technology to measure and record environmental data under the citizen science programs has greatly improved the speed, volume, and quality of data. Malthus et al. ( 2020 ) examined the impact of citizen science smartphone applications (Apps) on remotely sensed surface reflectance, stream sediment, and algal concentrations in 32 stream sites in eastern Australia. Smartphone Apps provide a friendly interface for citizen scientists to engage with and use sophisticated modern water quality monitoring technology. Smartphones are widely accessible, and the Apps are customised for objective, comprehensive, and accurate data capture (Pattinson et al. 2023 ).
Citizen science can complement microalgal biomonitoring of aquatic metal pollution in stream ecosystems through increased data collection. Citizen science projects engage a broader range of participants, allowing for more extensive data collection across various locations and times (Njue et al. 2019 ; Babiso et al. 2023 ). This can provide a more comprehensive understanding of the spatial and temporal dynamics of metal pollution in stream ecosystems. Furthermore, citizen science involves community engagement and creates social accountability and awareness towards environmental stewardship (Ruppen and Brugger 2022 ). Involving citizens in scientific monitoring fosters a sense of ownership and stewardship over local environments. This leads to increased awareness of environmental issues such as metal pollution and promotes sustainable behaviours to mitigate them. Citizen science, being a cost-effective metal pollution monitoring technique, can leverage the manpower and resources of volunteers, reducing the costs associated with monitoring efforts (Njue et al. 2019 ; Ruppen and Brugger 2022 ). This enables more frequent sampling and monitoring, which is essential for detecting changes in metal pollution levels over time. However, there is a need to identify and address the potential for errors and biases in integrating this approach in the biomonitoring of stream ecosystems (Follett and Strezov 2015 ).
Challenges and opportunities in integrating microalgae in aquatic biomonitoring of metal pollution
Despite the bottlenecks in the integrative monitoring of aquatic metal pollution in lotic systems of SSA using algal communities as bioindicators, several opportunities also present further room for developing a microalgae-based assessment of stream health status in the region. We highlight the challenges and opportunities for developing higher resolution, site-specific, and species-targeted microalgal-based bioassessment in SSA.
From our literature search, while other bioindicator taxa, particularly macroorganisms, are popular options, the use of algal communities to assess aquatic metal pollution, where this has been attempted, has been limited to the morphotaxonomic level. Besides not offering the benefit of higher resolution in detecting sub-lethal metal contamination, the absence of region-specific baseline data in several SSA sub-regions further limits the comprehensiveness of their use as bioindicators. Additionally, accurate identification of microalgal species requires trained morphotaxonomists and special equipment, such as the high-resolution scanning electron microscope, which may be limited in SSA. The uptake and integration of microalgae-based bioindicators into cutting-edge biomonitoring tools such as molecular (eRNA and eDNA), artificial intelligence systems (e.g., biosensors), and geospatial systems are yet to take off significantly. Accurate identification is essential for proper assessment and quantification of the magnitude of metal pollution and potential ecological risks to the provision of stream ecosystem services in SSA.
Microalgal communities and populations exhibit significant seasonal fluctuations, which must be understood to allow the partitioning of metal-pollution-induced impacts. Climate change, seasonality, and natural and anthropogenic factors influence microalgal community composition and species abundance. Seasonal fluctuations, such as variations in rainfall and temperature, significantly influence the hydrology of aquatic ecosystems, affecting the transport and deposition of metals (Maphanga et al. 2024 ). For instance, intense rainfall during the wet season can remobilise metals from soil and sediment into water bodies, causing elevated metal concentrations (Conrad et al. 2020 ). Conversely, dry seasons may concentrate metals due to decreased dilution and increased evaporation rates (Edokpayi et al. 2017 ). Extreme climatic events, such as floods and drought, further exacerbate metal pollution by altering metal transport and sedimentation patterns, which may introduce metals from repositories into water bodies (Xia et al. 2015 ; Wijngaard et al. 2017 ). Anthropogenic activities such as mining, industrial effluents, and agricultural runoff are significant sources of metal pollution in sub-Saharan Africa (Laffite et al. 2020 ). These activities introduce high concentrations of metals such as Pb, Hg, Cu, and Cd into aquatic ecosystems, which are highly toxic to aquatic biota (Hama Aziz et al. 2023 ; Fatmi et al. 2023 ). The interaction between seasonal dynamics, extreme climatic events, and anthropogenic activities underscores the complexity of integrating microalgae in monitoring aquatic metal pollution in SSA.
Furthermore, in the aquatic environment, metal species and mixtures interact differently with microalgae community species and vary in toxicity. Understanding these interactions and the ecological impact on microbial bioindicators, including microalgae, in the SSA is poorly understood due to the complexity and, hence, the need for cutting-edge research using advanced methods and sophisticated analytical equivalents. Unfortunately, many regions in SSA suffer from limited monitoring infrastructure, which hampers effective biomonitoring and data availability. Also, SSA, as a low to middle-income subregion, is marked by limited financial capacity to fully support advanced environmental stewardship programs in light of other “critical” financial obligations.
Opportunities
Similar to other tropical regions, SSA has a high diversity of microalgal communities and species richness due to its varied and extensively interconnected aquatic habitats. The biodiversity and richness can be leveraged to select the highly sensitive and most indicative species that respond specifically to individual and mixtures of aquatic metal pollution at sub-lethal concentrations. Since the most suitable microalgal communities and species have high sensitivity to changes in water quality, they can serve as potential early warning indicators of aquatic metal pollution episodes in river systems. Therefore, integrating microalgae in stream health assessment will improve early detection and further inform proactive interventions in managing stream ecosystems in the SSA mining regions.
Collaborative research within the SSA and with international research institutions and partners will address the limitations of access to advanced analytical tools, expertise, and limited funding. In particular, adopting modern research and monitoring tools such as eDNA and molecular biosensors will improve the resolution of detection of aquatic metal pollution. Establishing and expanding baseline data collection and accessible online databases will further accelerate the integration of microalgae for monitoring aquatic metal pollution in aquatic ecosystems across SSA.
Conclusion and future perspectives
Integrating microalgal communities as bioindicators of aquatic metal pollution in rivers of sub-Saharan Africa holds great promise for enhancing water quality monitoring and environmental conservation efforts. However, in the past decade and a half, the inclusion of microalgal taxa for integrative monitoring into aquatic metal pollution monitoring programs in the SSA has been low but gradually improving past 2020. The region is still lagging in the integration of emerging tools, such as environmental DNA, and technological advances, such as artificial intelligence models, remote sensing, and citizen science, that offer potential benefits of high precision, speed, reduced costs, and eco-friendly green technologies in monitoring and assessment of stream ecosystem health across its mining landscapes.
Despite the lack of a standardised, synchronised, and adequately documented microalgal database, inadequate microalgal taxonomic and molecular assessment expertise, limited monitoring and processing infrastructure, and economic constraints, the integrative microalgae-based approach offers significant opportunities for addressing aquatic metal pollution in the SSA lotic ecosystems. The high biodiversity of microalgae in SSA presents a vast pool for selecting suitable site-specific (sensitive and indicative) taxa that respond specifically to individual and mixtures of metal pollutants. Different microalgal taxa have been combined with other bioindicator groups to increase the sensitivity to pollutant detection source tracking and quantification. This uniqueness emphasises the central role of microalgae in aquatic ecosystem biomonitoring initiatives. Moreover, the rapid response to changes in water quality during integrative monitoring positions microalgae as potential early warning indicators for aquatic metal pollution events that impact aquatic ecosystems.
Fundamentally, there is an urgent need to prioritise efforts to institutionalism and strengthen and standardise national and regional baseline data collection on microalgae dynamics in response to metal pollution in aquatic ecosystems across SSA. Such data will serve as a foundation for accuracy and a reference point for improving the assessments of metal pollution and ecological impacts on the region’s aquatic ecosystems.
Collaboration among regional and international research institutions and organisations can lead to the development of integrated monitoring networks. These networks can leverage advanced analytical tools, technologies, and expertise to enhance data collection and analysis. Furthermore, involving riparian communities in data collection and monitoring efforts fosters a sense of collective responsibility and ownership of the stream ecosystem resources. In fact, “citizen science” initiatives are crucial to empowering riparian communities to participate actively in the conservation of stream ecosystems.
Continued research into the interactions between metal pollutants and microalgal species is essential for better understanding the ecological consequences of aquatic metal pollution. Complementing conventional monitoring with innovative techniques, such as artificial intelligence, molecular tools, and remote sensing, must be prioritised to improve the overall efficiency and maximise the productivity of environmental stewardship in metal mining regions.
Data availability
All the data generated are available in this review. Additional data and information can be sourced from the cited references and online databases or sources.
Abdel-Karim R (2024) Nanotechnology-enabled biosensors: a review of fundamentals, materials, applications, challenges, and future scope. Biomed Mater Devices. https://doi.org/10.1007/s44174-023-00147-z
Article Google Scholar
Addo-Bediako A, Nukeri S, Kekana M (2021) Heavy metal and metalloid contamination in the sediments of the Spekboom River, South Africa. Appl Water Sci 11:133. https://doi.org/10.1007/s13201-021-01464-8
Alfadaly RA, Elsayed A, Hassan RYA et al (2021) Microbial sensing and removal of heavy metals: bioelectrochemical detection and removal of chromium(VI) and cadmium(II). Molecules 26:2549. https://doi.org/10.3390/molecules26092549
Article CAS Google Scholar
Ali GH, Abd el-Salam NF (1999) Factors controlling bioindicators for industrial pollution detection. Biomed Environ Sci 12:194–200
CAS Google Scholar
Ali H, Khan E (2019) Trophic transfer, bioaccumulation, and biomagnification of non-essential hazardous heavy metals and metalloids in food chains/webs—concepts and implications for wildlife and human health. Hum Ecol Risk Assess an Int J 25:1353–1376. https://doi.org/10.1080/10807039.2018.1469398
Amoatey P, Baawain MS (2019) Effects of pollution on freshwater aquatic organisms. Water Environ Res 91:1272–1287. https://doi.org/10.1002/wer.1221
Ancion P-Y, Lear G, Lewis GD (2010) Three common metal contaminants of urban runoff (Zn, Cu & Pb) accumulate in freshwater biofilm and modify embedded bacterial communities. Environ Pollut 158:2738–2745. https://doi.org/10.1016/j.envpol.2010.04.013
Apothéloz-Perret-Gentil L, Bouchez A, Cordier T et al (2021) Monitoring the ecological status of rivers with diatom eDNA metabarcoding: a comparison of taxonomic markers and analytical approaches for the inference of a molecular diatom index. Mol Ecol 30:2959–2968. https://doi.org/10.1111/mec.15646
Astatkie H, Ambelu A, Mengistie E (2021) Contamination of stream sediment with heavy metals in the Awetu watershed of Southwestern Ethiopia. Front Earth Sci 9:1–13. https://doi.org/10.3389/feart.2021.658737
Avtar R, Komolafe AA, Kouser A et al (2020) Assessing sustainable development prospects through remote sensing: a review. Remote Sens Appl Soc Environ 20:100402. https://doi.org/10.1016/j.rsase.2020.100402
Awofolu OR (2005) Determination and seasonal variation of heavy metals in algae and sediments in sewers from industrial areas in Lagos State, Nigeria. Pak J Sci Ind Res 48:28–36
Babiso WZ, Ayano KK, Haile AT et al (2023) Citizen science for water quality monitoring in the Meki River, Ethiopia: quality assurance and comparison with conventional methods. Water 15:238. https://doi.org/10.3390/w15020238
Bae M-J, Park Y-S (2014) Biological early warning system based on the responses of aquatic organisms to disturbances: A review. Sci Total Environ 466–467:635–649. https://doi.org/10.1016/j.scitotenv.2013.07.075
Banze wa Mutombo A, Atibu EK, Mbuya wa Mutombo J et al (2022) Contamination by heavy metals from mining activities: an ecological impact assessment of Mura and Kimpulande Rivers, Democratic Republic of the Congo. Watershed Ecol Environ 4:148–157. https://doi.org/10.1016/j.wsee.2022.10.004
Bashir I, Lone FA, Bhat RA et al (2020) Concerns and threats of contamination on aquatic ecosystems. Bioremediation and biotechnology. Springer International Publishing, Cham, pp 1–26
Google Scholar
Beecham S, Baddoo N, Hall T et al (2008) Motivation in software engineering: a systematic literature review. Inf Softw Technol 50:860–878. https://doi.org/10.1016/j.infsof.2007.09.004
Bellinger EG, Sigee DC (2015) Fresh water algae: indentification, enumeration and use as bioincators, 2nd edn. John Wiley & Sons, Chichester
Book Google Scholar
Beng KC, Corlett RT (2020) Applications of environmental DNA (eDNA) in ecology and conservation: opportunities, challenges and prospects. Biodivers Conserv 29:2089–2121. https://doi.org/10.1007/s10531-020-01980-0
Bere T, Mangadze T, Mwedzi T (2016) Variation partitioning of diatom species data matrices: understanding the influence of multiple factors on benthic diatom communities in tropical streams. Sci Total Environ 566–567:1604–1613. https://doi.org/10.1016/j.scitotenv.2016.06.058
Bermanec V, Palinkaš LA, Fiket Ž et al (2018) Interaction of acid mine drainage with biota in the Allchar Carlin-type As-Tl-Sb-Au deposit, Macedonia. J Geochemical Explor 194:104–119. https://doi.org/10.1016/j.gexplo.2018.07.015
Beyene A, Addis T, Kifle D et al (2009) Comparative study of diatoms and macroinvertebrates as indicators of severe water pollution: case study of the Kebena and Akaki rivers in Addis Ababa, Ethiopia. Ecol Indic 9:381–392. https://doi.org/10.1016/j.ecolind.2008.05.001
Biney C, Amuzu ATT, Calamari D et al (1994) Review of heavy metals in the African aquatic environment. Ecotoxicol Environ Saf 28:134–159. https://doi.org/10.1006/eesa.1994.1041
Blanco S, Bécares E (2010) Are biotic indices sensitive to river toxicants? A comparison of metrics based on diatoms and macro-invertebrates. Chemosphere 79:18–25. https://doi.org/10.1016/j.chemosphere.2010.01.059
Borgwardt F, Robinson L, Trauner D et al (2019) Exploring variability in environmental impact risk from human activities across aquatic ecosystems. Sci Total Environ 652:1396–1408. https://doi.org/10.1016/j.scitotenv.2018.10.339
Bresciani M, Giardino C, Lauceri R et al (2016) Earth observation for monitoring and mapping of cyanobacteria blooms. Case studies on five Italian lakes. J Limnol 76. https://doi.org/10.4081/jlimnol.2016.1565
Bunholi IV, Foster NR, Casey JM (2023) Environmental DNA and RNA in aquatic community ecology: toward methodological standardization. Environ DNA 5:1133–1147. https://doi.org/10.1002/edn3.476
Burada A, Ţopa CM, Georgescu LP et al (2015) Heavy metals accumulation in plankton and water of four aquatic complexes from Danube Delta area. Aquac Aquarium Conserv Legis Int J Bioflux Soc 7:301–310
Calmuc VA, Calmuc M, Arseni M et al (2021) Assessment of heavy metal pollution levels in sediments and of ecological risk by quality indices, applying a case study: the lower Danube River, Romania. Water 13:1801. https://doi.org/10.3390/w13131801
Cao Y, Ye Y, Zhao H et al (2018) Remote sensing of water quality based on HJ-1A HSI imagery with modified discrete binary particle swarm optimization-partial least squares (MDBPSO-PLS) in inland waters: a case in Weishan Lake. Ecol Inform 44:21–32. https://doi.org/10.1016/j.ecoinf.2018.01.004
Cerminati S, Soncini FC, Checa SK (2015) A sensitive whole-cell biosensor for the simultaneous detection of a broad-spectrum of toxic heavy metal ions. Chem Commun 51:5917–5920. https://doi.org/10.1039/c5cc00981b
Chakraborty P, Raghunadh Babu PV, Acharyya T, Bandyopadhyay D (2010) Stress and toxicity of biologically important transition metals (Co, Ni, Cu and Zn) on phytoplankton in a tropical freshwater system: an investigation with pigment analysis by HPLC. Chemosphere 80:548–553. https://doi.org/10.1016/j.chemosphere.2010.04.039
Chalumba KS, Eliezer MB, Patricia B et al (2021) Assessment of heavy metals in wild and farmed tilapia (Oreochromis niloticus) on Lake Kariba, Zambia: implications for human and fish health. Food Addit Contam Part A 39:74–91. https://doi.org/10.1080/19440049.2021.1975830
Chetty S, Pillay L (2019) Assessing the influence of human activities on river health: a case for two South African rivers with differing pollutant sources. Environ Monit Assess 191:168. https://doi.org/10.1007/s10661-019-7308-4
Chi G, Ma J, Shi Y, Chen X (2016) Hyperspectral remote sensing of cyanobacterial pigments as indicators of the iron nutritional status of cyanobacteria-dominant algal blooms in eutrophic lakes. Ecol Indic 71:609–617. https://doi.org/10.1016/j.ecolind.2016.06.014
Chmielewská E, Medved’ J (2001) Bioaccumulation of heavy metals by green algae Cladophora gramerata in a refiner sewage lagoon. Croat Chem Acta 74:135–145
Chon T-S, Qu X, Cho W-S et al (2013) Evaluation of stream ecosystem health and species association based on multi-taxa (benthic macroinvertebrates, algae, and microorganisms) patterning with different levels of pollution. Ecol Inform 17:58–72. https://doi.org/10.1016/j.ecoinf.2013.06.004
Cid A, Prado R, Rioboo C et al (2012) Use of microalgae as biological indicators of pollution: looking for new relevant cytotoxicity endpoints. Microalgae: biotechnology, microbiology and energy. Nova Science Publishers, New York, pp 311–323
Cilleros K, Valentini A, Allard L et al (2019) Unlocking biodiversity and conservation studies in high-diversity environments using environmental DNA (eDNA): a test with Guianese freshwater fishes. Mol Ecol Resour 19:27–46. https://doi.org/10.1111/1755-0998.12900
Conrad SR, Santos IR, White SA et al (2020) Elevated dissolved heavy metal discharge following rainfall downstream of intensive horticulture. Appl Geochem 113:104490. https://doi.org/10.1016/j.apgeochem.2019.104490
Cook KV, Beyer JE, Xiao X, Hambright KD (2023) Ground-based remote sensing provides alternative to satellites for monitoring cyanobacteria in small lakes. Water Res 242:120076. https://doi.org/10.1016/j.watres.2023.120076
Cormier R, Elliott M, Rice J (2019) Putting on a bow-tie to sort out who does what and why in the complex arena of marine policy and management. Sci Total Environ 648:293–305. https://doi.org/10.1016/j.scitotenv.2018.08.168
Cornelissen B, Zaidman A, van Deursen A et al (2009) A systematic survey of program comprehension through dynamic analysis. IEEE Trans Softw Eng 35:684–702. https://doi.org/10.1109/TSE.2009.28
Costanza R, De GR, Sutton P et al (2014) Changes in the global value of ecosystem services. Glob Environ Chang 26:152–158. https://doi.org/10.1016/j.gloenvcha.2014.04.002
Culhane F, Teixeira H, Nogueira AJA et al (2019) Environment risk to the supply of ecosystem services across aquatic ecosystems. Sci Total Environ 660:611–621. https://doi.org/10.1016/j.scitotenv.2018.12.346
Dalu T, Froneman PW, Richoux NB (2014) Phytoplankton community diversity along a river-estuary continuum. Trans R Soc South Africa 69:107–116. https://doi.org/10.1080/0035919X.2014.930074
Dalu T, Wasserman RJ, Magoro ML et al (2017) Variation partitioning of benthic diatom community matrices: effects of multiple variables on benthic diatom communities in an Austral temperate river system. Sci Total Environ 601–602:73–82. https://doi.org/10.1016/j.scitotenv.2017.05.162
Dalu T, Cuthbert RN, Weyl OLF, Wasserman RJ (2022a) Community structure and environmental factors affecting diatom abundance and diversity in a Mediterranean climate river system. Sci Total Environ 810:152366. https://doi.org/10.1016/j.scitotenv.2021.152366
Dalu T, Mwedzi T, Wasserman RJ et al (2022b) Land use effects on water quality, habitat, and macroinvertebrate and diatom communities in African highland streams. Sci Total Environ 846:157346. https://doi.org/10.1016/j.scitotenv.2022.157346
Deiner K, Fronhofer EA, Mächler E et al (2016) Environmental DNA reveals that rivers are conveyer belts of biodiversity information. Nat Commun 7:12544. https://doi.org/10.1038/ncomms12544
Deng P, Lei Y, Liu W, Wang X (2012) Exploration of benthic diatom indices to evaluate water quality in rivers in the Dongjiang basin. Shengtai Xuebao/ Acta Ecol Sin 32:5014–5024. https://doi.org/10.5846/stxb201112071867
Dokulil MT, Donabaum U, Vienna LS (2016) Phytoplankton of the Danube River : composition and long-term dynamics. Acta Zool Bulg 7:147–152
Dora SL, Chowdhury A, Maiti SK, Tiwary RK (2021) Assessment of pollution load and identifying bioindicator algal species using multivariate statistical techniques: a case study from Damodar River, India. Int J Environ Pollut 69:151. https://doi.org/10.1504/IJEP.2021.126971
Edokpayi JN, Odiyo JO, Popoola EO, Msagati TAM (2017) Evaluation of temporary seasonal variation of heavy metals and their potential ecological risk in Nzhelele River, South Africa. Open Chem 15:272–282. https://doi.org/10.1515/chem-2017-0033
Ezewudo BI, Mgbenka BO, Islam S, Odo GE (2021) Appraisal of metal contamination in sediments of lower reaches of Niger River, Nigeria, using contamination indices and sediment quality guidelines. Int J Environ Anal Chem 00:1–20. https://doi.org/10.1080/03067319.2021.1895140
Fatmi B, Hazzab A, Ghenaim A, Rahmani A (2023) anthropogenic pollution and ecological risk in urban sediments: assessing heavy metal and organic contamination in the Saida watershed, North-Western Algeria. Water Air Soil Pollut 234:699. https://doi.org/10.1007/s11270-023-06701-1
Fayiga AO, Abioye O, Ipinmoroti MO et al (2018) Environmental pollution in Africa. Environ Dev Sustain 20:41–73. https://doi.org/10.1007/s10668-016-9894-4
Feisal NAS, Kamaludin NH, Abdullah Sani MF et al (2023) Anthropogenic disturbance of aquatic biodiversity and water quality of an urban river in Penang, Malaysia. Water Sci Eng 16:234–242. https://doi.org/10.1016/j.wse.2023.01.003
Feng B, Zhang M, Chen J et al (2021) Reduction in the phytoplankton index of biotic integrity in riverine ecosystems driven by industrial activities, dam construction and mining: a case study in the Ganjiang River, China. Ecol Indic 120:106907. https://doi.org/10.1016/j.ecolind.2020.106907
Ferreira V, Albariño R, Larrañaga A et al (2023) Ecosystem services provided by small streams: an overview. Hydrobiologia 850:2501–2535. https://doi.org/10.1007/s10750-022-05095-1
Ferreira da Silva E, Almeida SFPP, Nunes ML et al (2009) Heavy metal pollution downstream the abandoned Coval da Mó mine (Portugal) and associated effects on epilithic diatom communities. Sci Total Environ 407:5620–5636. https://doi.org/10.1016/j.scitotenv.2009.06.047
Follett R, Strezov V (2015) An analysis of citizen science based research: usage and publication patterns. PLoS One 10:e0143687. https://doi.org/10.1371/journal.pone.0143687
Forio MAE, Goethals PLM (2020) An integrated approach of multi-community monitoring and assessment of aquatic ecosystems to support sustainable development. Sustainability 12:5603. https://doi.org/10.3390/su12145603
Fraisl D, Hager G, Bedessem B et al (2022) Citizen science in environmental and ecological sciences. Nat Rev Methods Prim 2:64. https://doi.org/10.1038/s43586-022-00144-4
Gbogbo F, Otoo SD (2015) The concentrations of five heavy metals in components of an economically important urban coastal wetland in Ghana: public health and phytoremediation implications. Environ Monit Assess 187:655. https://doi.org/10.1007/s10661-015-4880-0
Gerber R, Smit NJ, van Vuren JHJ et al (2015) Application of a sediment quality index for the assessment and monitoring of metals and organochlorines in a premier conservation area. Environ Sci Pollut Res 22:19971–19989. https://doi.org/10.1007/s11356-015-5206-z
Gleason JE, Elbrecht V, Braukmann TWA et al (2021) Assessment of stream macroinvertebrate communities with eDNA is not congruent with tissue-based metabarcoding. Mol Ecol 30:3239–3251. https://doi.org/10.1111/mec.15597
Guo Y, Liang Y, Deng R et al (2022) Development and application of a new sensitivity analysis model for the remote sensing retrieval of heavy metals in water. Heliyon 8:e12033. https://doi.org/10.1016/j.heliyon.2022.e12033
Hama Aziz KH, Mustafa FS, Omer KM et al (2023) Heavy metal pollution in the aquatic environment: efficient and low-cost removal approaches to eliminate their toxicity: a review. RSC Adv 13:17595–17610. https://doi.org/10.1039/d3ra00723e
Hamza-Chaffai A (2014) Usefulness of bioindicators and biomarkers in pollution biomonitoring. Int J Biotechnol Wellness Ind 3:19–26. https://doi.org/10.6000/1927-3037.2014.03.01.4
Hansen BK, Jacobsen MW, Middelboe AL et al (2020) Remote, autonomous real-time monitoring of environmental DNA from commercial fish. Sci Rep 10:13272. https://doi.org/10.1038/s41598-020-70206-8
Harding B, Taylor JC (2014) Diatoms as indicators of historical water quality: a comparison of samples taken in the Wemmershoek catchment (Western Province, South Africa) in 1960 and 2008. Water SA 40:601–606. https://doi.org/10.4314/wsa.v40i4.4
Harrison JB, Sunday JM, Rogers SM (2019) Predicting the fate of eDNA in the environment and implications for studying biodiversity. Proc R Soc B Biol Sci 286:20191409. https://doi.org/10.1098/rspb.2019.1409
Hattikudur RS, Alakananda B, Ramachandra TV (2014) Diatoms as bioindicators. In: LAKE 2014: Conference on Conservation and Sustainable Management of Wetland Ecosystems in Western Ghats, 13–15 November, 2014. Sirsi, India, pp 2012–2015
Hena JS, Magaji JI, Kulawe D (2022) Dsitribution of heavy metal and phytoplankton in Calabar River port terminals, Calabar, Cross River state, Nigeria. Sci World J 17:521–525
Huang X, Zhu Y, Kianfar E (2021) Nano biosensors: properties, applications and electrochemical techniques. J Mater Res Technol 12:1649–1672. https://doi.org/10.1016/j.jmrt.2021.03.048
Huang CW, Lin C, Nguyen MK et al (2023) A review of biosensor for environmental monitoring: principle, application, and corresponding achievement of sustainable development goals. Bioengineered 14:58–80. https://doi.org/10.1080/21655979.2022.2095089
Ismail MM, El Zokm GM (2023) Evaluation of the response of phytoplankton communities to heavy metal stresses using multi-statistical approaches, Alexandria coast, Egypt. Int J Environ Sci Technol. https://doi.org/10.1007/s13762-023-04914-9
Jeon Y, Lee Y, Jang G et al (2022) Design of Pb(II)-Specific E. coli-based biosensors by engineering regulatory proteins and host cells. Front Microbiol 13:1–12. https://doi.org/10.3389/fmicb.2022.881050
Jordaan K, Comeau AM, Khasa DP, Bezuidenhout CC (2019) An integrated insight into the response of bacterial communities to anthropogenic contaminants in a river: a case study of the Wonderfonteinspruit catchment area, South Africa. Plos One 14:e0216758. https://doi.org/10.1371/journal.pone.0216758
Kaonga CC, Chiotha SS, Monjerezi M et al (2008) Levels of cadmium, manganese and lead in water and algae; Spirogyra aequinoctialis. Int J Environ Sci Technol 5:471–478. https://doi.org/10.1007/BF03326043
Kayembe JM, Sivalingam P, Salgado CD et al (2018) Assessment of water quality and time accumulation of heavy metals in the sediments of tropical urban rivers: Case of Bumbu River and Kokolo Canal, Kinshasa City, Democratic Republic of the Congo. J African Earth Sci 147:536–543. https://doi.org/10.1016/j.jafrearsci.2018.07.016
Keck F, Vasselon V, Rimet F et al (2018) Boosting DNA metabarcoding for biomonitoring with phylogenetic estimation of operational taxonomic units’ ecological profiles. Mol Ecol Resour 18:1299–1309. https://doi.org/10.1111/1755-0998.12919
Khatri N, Tyagi S (2015) Influences of natural and anthropogenic factors on surface and groundwater quality in rural and urban areas. Front Life Sci 8:23–39. https://doi.org/10.1080/21553769.2014.933716
Kimirei IA, Mubaya CP, Ndebele-Murisa M et al (2021) Trends in ecological changes. In: Ndebele-Murisa M, Kimirei IA, Mubaya CP, Bere T (eds) Ecological changes in the Zambezi River Basin. CODESRIA, Dakar, Senegal, pp 49–82. https://doi.org/10.2307/j.ctv1grbbq5.10
Kitchenham B, Charters S (2007) Guidelines for performing systematic literature reviews in software engineering. EBSE Technical Report, EBSE-2007-01. Durham, UK
Kock A, Taylor JC, Malherbe W (2019) Diatom community structure and relationship with water quality in Lake Sibaya, KwaZulu-Natal, South Africa. South Afr J Bot 123:161–169. https://doi.org/10.1016/j.sajb.2019.03.013
Kumari D, Paul DK (2020) Assessing the role of bioindicators in freshwater ecosystem. J Interdiscipl Cycle Res 12:58–74
Laffite A, Al Salah DMM, Slaveykova VI et al (2020) Impact of anthropogenic activities on the occurrence and distribution of toxic metals, extending-spectra β-lactamases and carbapenem resistance in sub-Saharan African urban rivers. Sci Total Environ 727:138129. https://doi.org/10.1016/j.scitotenv.2020.138129
Lamare M (2019) Plankton: a guide to their ecology and monitoring for water quality, 2nd edition. New Zeal J Mar Freshw Res 53:480–480. https://doi.org/10.1080/00288330.2019.1625497
Lang P, Taylor JCC, Bertolli L et al (2013) Proposed procedure for the sampling, preparation and analysis of benthic diatoms from Zambian rivers: a bioassessment and decision support tool applicable to freshwater ecoregions in tropical southern Africa. SAFRASS Project (AFS/2009/219013) Report. East Kilbride, Scotland
Laporte M, Berger CS, García-Machado E et al (2022) Cage transplant experiment shows weak transport effect on relative abundance of fish community composition as revealed by eDNA metabarcoding. Ecol Indic 137:0–7. https://doi.org/10.1016/j.ecolind.2022.108785
Lavoie I, Lavoie M, Fortin C (2012) A mine of information: benthic algal communities as biomonitors of metal contamination from abandoned tailings. Sci Total Environ 425:231–241. https://doi.org/10.1016/j.scitotenv.2012.02.057
Lazorchak JM, Hill BH, Brown BS et al (2003) USEPA biomonitoring and bionindicator concepts needed to evaluate the biological integrity of aquatic ecosystems. In: Markert BA, Breure AM, Zechmeister HG (eds) Bioindicators & biomonitors : principles, concepts, and applications, 1st edn. Elsevier Science Ltd, New York, pp 123–152
Lee K-L, Choi JS, Lee J-H et al (2021) Response of epilithic diatom assemblages to weir construction on the Nakdong River, Republic of Korea. Ecol Indic 126:107711. https://doi.org/10.1016/j.ecolind.2021.107711
Leguay S, Lavoie I, Levy JL, Fortin C (2016) Using biofilms for monitoring metal contamination in lotic ecosystems: the protective effects of hardness and pH on metal bioaccumulation. Environ Toxicol Chem 35:1489–1501. https://doi.org/10.1002/etc.3292
Lemley DA, Adams JB, Bate GC (2016) A review of microalae as indicators in South African estuaries. South Afr J Bot 107:12–20. https://doi.org/10.1016/j.sajb.2016.04.008
Li L, Zheng B, Liu L et al (2010) Metagenomic applications in environmental monitoring and bioremediation. J Ind Microbiol Biotechnol 43:1345–1354. https://doi.org/10.1016/j.tibtech.2010.03.005
Li F, Peng Y, Fang W et al (2018) Application of environmental DNA metabarcoding for predicting anthropogenic pollution in rivers. Environ Sci Technol 52:11708–11719. https://doi.org/10.1021/acs.est.8b03869
Li Y, Zhou Q, Zhang Y et al (2021) Research trends in the remote sensing of phytoplankton blooms: results from bibliometrics. Remote Sens 13:4414. https://doi.org/10.3390/rs13214414
Lin Y, Gao J, Tu Y et al (2024) Estimating low concentration heavy metals in water through hyperspectral analysis and genetic algorithm-partial least squares regression. Sci Total Environ 916:170225. https://doi.org/10.1016/j.scitotenv.2024.170225
Limburg KE (2009) Aquatic ecosystem services. In: Likens GE (ed) Encyclopedia of inland waters, 1st edn. Elsevier, Millbrook, pp 25–30. https://doi.org/10.1016/B978-012370626-3.00004-1
Limburg KE, Swaney DP, Strayer DL (2013) River ecosystems. In: Levin SA (ed) Encyclopedia of biodiversity, 2nd edn. Elsevier, Millbrook, pp 469–484. https://doi.org/10.1016/B978-0-12-384719-5.00222-7
Lobo EA, Heinrich CG, Schuch M et al (2016) Diatoms as bioindicators in rivers. River algae. Springer International Publishing, Cham, pp 245–271
Chapter Google Scholar
Maes J, Liquete C, Teller A et al (2020) An indicator framework for assessing ecosystem services in support of the EU biodiversity strategy to 2020. 17:14–23. https://doi.org/10.1016/j.ecoser.2015.10.023
Malthus TJ, Ohmsen R, Woerd HJ van der (2020) An evaluation of citizen science smartphone apps for inland water quality assessment. Remote Sens 12:1578. https://doi.org/10.3390/rs12101578
Mangadze T, Bere T, Mwedzi T (2016) Choice of biota in stream assessment and monitoring programs in tropical streams: a comparison of diatoms, macroinvertebrates and fish. Ecol Indic 63:128–143. https://doi.org/10.1016/j.ecolind.2015.11.029
Mangadze T, Wasserman RJ, Dalu T (2017) Use of diatom communities as indicators of conductivity and ionic composition in a small austral temperate river system. Water Air Soil Pollut 228:428. https://doi.org/10.1007/s11270-017-3610-3
Mangadze T, Dalu T, William Froneman P (2019a) Biological monitoring in southern Africa: a review of the current status, challenges and future prospects. Sci Total Environ 648:1492–1499. https://doi.org/10.1016/j.scitotenv.2018.08.252
Mangadze T, Taylor JC, Froneman WP, Dalu T (2019b) Water quality assessment in a small austral temperate river system (Bloukrans River system, South Africa): application of multivariate analysis and diatom indices. South Afr J Bot 125:353–359. https://doi.org/10.1016/j.sajb.2019.08.008
Maphanga T, Chidi BS, Phungela TT et al (2024) The interplay between temporal and seasonal distribution of heavy metals and physiochemical properties in Kaap River. Int J Environ Sci Technol 21:6053–6064. https://doi.org/10.1007/s13762-023-05401-x
Marcel R, Berthon V, Castets V, et al (2017) Modelling diatom life forms and ecological guilds for river biomonitoring. Knowl Manag Aquat Ecosyst 1. https://doi.org/10.1051/kmae/2016033
Marques JA, Costa SR, Maraschi AC et al (2022) Biochemical response and metals bioaccumulation in planktonic communities from marine areas impacted by the Fundão mine dam rupture (southeast Brazil). Sci Total Environ 806:150727. https://doi.org/10.1016/j.scitotenv.2021.150727
Masouras A, Karaouzas I, Dimitriou E et al (2021) Benthic diatoms in river biomonitoring-present and future perspectives within the water framework directive. Water (Switzerland) 13. https://doi.org/10.3390/w13040478
Mccabe DJ (2010) Rivers and streams: life in flowing water streams and rivers: habitats partitioned at different spatial scales. Nat Educ Knowl 1:1–14
MEA (2005) Ecosystems and human well-being: opportunities for business and industry. A report of the millennium ecosystem assessment. Island Press, Washington, DC
Miguel-Chinchilla L, Heasley E, Loiselle S, Thornhill I (2019) Local and landscape influences on turbidity in urban streams: a global approach using citizen scientists. Freshw Sci 38:303–320. https://doi.org/10.1086/703460
Mishra S, Bharagava RN, More N et al (2019) Heavy metal contamination: an alarming threat to environment and human health. In: Sobti RC, Arora NK, Kothari R (eds) Environmental biotechnology: for sustainable future. Springer, Singapore, pp 103–125. https://doi.org/10.1007/978-981-10-7284-0_5
Mohajane C, Manjoro M (2022) Sediment-associated heavy metal contamination and potential ecological risk along an urban river in South Africa. Heliyon 8:e12499. https://doi.org/10.1016/j.heliyon.2022.e12499
Mohammed AH, Khalifa AM, Mohamed HM et al (2024) Assessment of heavy metals at mangrove ecosystem, applying multiple approaches using in-situ and remote sensing techniques, Red Sea, Egypt. Environ Sci Pollut Res 31:8118–8133. https://doi.org/10.1007/s11356-023-31625-y
Moharana T (2021) The Science of Citizen Science. Proc Nov Scotian Inst Sci 51:4. https://doi.org/10.15273/pnsis.v51i2.11167
Moyo S, McCrindle R, Mokgalaka N, Myburgh J (2015) Heavy metal partitioning in sediments from rivers flowing through coal fields in Mpumalanga, South Africa. CLEAN - Soil, Air, Water 43:892–900. https://doi.org/10.1002/clen.201300656
Mwedzi T, Siziba N, Odume ON et al (2020) Responses of macroinvertebrate community metrics to urban pollution in semi-arid catchments around the city of Bulawayo, Zimbabwe. Water SA 46:583–592. https://doi.org/10.17159/wsa/2020.v46.i4.9071
Ngueyep MLL, Kingni KS, Ngounouno NM et al (2021) The impact of gold mining exploitation on the physicochemical quality of water: case of Batouri (Cameroon). Int J Energy Water Resour 5:159–173. https://doi.org/10.1007/s42108-020-00106-0
Nguyen TH, Helm B, Hettiarachchi H et al (2019) The selection of design methods for river water quality monitoring networks: a review. Environ Earth Sci 78:96. https://doi.org/10.1007/s12665-019-8110-x
Ngure V, Davies T, Kinuthia G et al (2014) Concentration levels of potentially harmful elements from gold mining in Lake Victoria Region, Kenya: environmental and health implications. J Geochem Explor 144:511–516. https://doi.org/10.1016/j.gexplo.2014.04.004
Njue N, Stenfert Kroese J, Gräf J et al (2019) Citizen science in hydrological monitoring and ecosystem services management: state of the art and future prospects. Sci Total Environ 693:133531. https://doi.org/10.1016/j.scitotenv.2019.07.337
Nkinda MS, Rwiza MJ, Ijumba JN, Njau KN (2020) Quantitative assessment of metal contamination and associated pollution risk in sediments from the Mara River in Tanzania. Environ Monit Assess 192:721. https://doi.org/10.1007/s10661-020-08681-9
Norros V, Laamanen T, Meissner K et al (2022) Roadmap for implementing environmental DNA (eDNA) and other molecular monitoring methods in Finland. Reports of the Finnish environment institute. Finnish Environment Institute, Helsinki
Nourmohammadi E, Hosseinkhani S, Nedaeinia R et al (2020) Construction of a sensitive and specific lead biosensor using a genetically engineered bacterial system with a luciferase gene reporter controlled by pbr and cadA promoters. Biomed Eng Online 19:1–13. https://doi.org/10.1186/s12938-020-00816-w
Oberholster PJ, De Klerk AR, De Klerk L et al (2016) Algal assemblage responses to acid mine drainage and steel plant wastewater effluent up and downstream of pre and post wetland rehabilitation. Ecol Indic 62:106–116. https://doi.org/10.1016/j.ecolind.2015.11.025
Oberholster PJ, Botha A-M, Hill L, Strydom WF (2017) River catchment responses to anthropogenic acidification in relationship with sewage effluent: an ecotoxicology screening application. Chemosphere 189:407–417. https://doi.org/10.1016/j.chemosphere.2017.09.084
Ochieng EZ, Lalah JO, Wandiga SO (2009) Anthropogenic sources of heavy metals in the Indian Ocean coast of Kenya. Bull Environ Contam Toxicol 83:600–607. https://doi.org/10.1007/s00128-009-9807-4
Ogoyi DO, Mwita CJJ, Nguu EK, Shiundu PM (2011) Determination of heavy metal content in water, sediment and microalgae from Lake Victoria, East Africa. Open Environ Eng J 4:156–161. https://doi.org/10.2174/1874829501104010156
Omara T, Karungi S, Kalukusu R et al (2019) Mercuric pollution of surface water, superficial sediments, Nile tilapia (Oreochromis nilotica Linnaeus 1758 [Cichlidae]) and yams ( Dioscorea alata) in auriferous areas of Namukombe stream, Syanyonja, Busia, Uganda. PeerJ 7:e7919. https://doi.org/10.7717/peerj.7919
Ouma KO, Shane A, Syampungani S (2022) Aquatic ecological risk of heavy-metal pollution associated with degraded mining landscapes of the Southern Africa River Basins: a review. Minerals 12:225. https://doi.org/10.3390/min12020225
Page MJ, McKenzie JE, Bossuyt PM et al (2021) The PRISMA 2020 statement: an updated guideline for reporting systematic reviews. PLOS Med 18:e1003583. https://doi.org/10.1371/journal.pmed.1003583
Pandey LK (2020) In situ assessment of metal toxicity in riverine periphytic algae as a tool for biomonitoring of fluvial ecosystems. Environ Technol Innov 18:100675. https://doi.org/10.1016/j.eti.2020.100675
Pandey LK, Bergey EA (2018) Metal toxicity and recovery response of riverine periphytic algae. Sci Total Environ 642:1020–1031. https://doi.org/10.1016/j.scitotenv.2018.06.069
Parmar TK, Rawtani D, Agrawal YK (2016) Bioindicators: the natural indicator of environmental pollution. Front Life Sci 9:110–118. https://doi.org/10.1080/21553769.2016.1162753
Pascale BM (2023) Spatio-temporal distribution of phytoplankton and water quality of some rivers of Haute Sanaga department ( Central Africa ). Biol LIfe Sci 14:1–18. https://doi.org/10.5296/jbls.v14i1.20181
Pereira-da-Conceicoa L, Elbrecht V, Hall A et al (2021) Metabarcoding unsorted kick-samples facilitates macroinvertebrate-based biomonitoring with increased taxonomic resolution, while outperforming environmental DNA. Environ DNA 3:353–371. https://doi.org/10.1002/edn3.116
Pattinson NB, Taylor J, Dickens CWS, Graham PM (2023) Digital innovation in citizen science to enhance water quality monitoring in developing countries. Colombo, Sri Lanka: International Water Management Institute (IWMI). 37. (IWMI working paper 210). https://doi.org/10.5337/2024.201
Perry I, Jâms IB, Casas-Mulet R et al (2022) Challenges to implementing environmental-DNA monitoring in Namibia. Front Environ Sci 9:773991. https://doi.org/10.3389/fenvs.2021.773991
Petticrew M, Roberts H (2008) Systematic reviews in the social sciences: a practical guide. Blackwell Publishing, Oxford. https://doi.org/10.1002/9780470754887
Pettorelli N, Schulte to Bühne H, Tulloch A et al (2018) Satellite remote sensing of ecosystem functions: opportunities, challenges and way forward. Remote Sens Ecol Conserv 4:71–93. https://doi.org/10.1002/rse2.59
Rajfur M, Kłos A, Wacławek M (2010) Sorption properties of algae Spirogyra sp. and their use for determination of heavy metal ions concentrations in surface water. Bioelectrochemistry 80:81–86. https://doi.org/10.1016/j.bioelechem.2010.03.005
Ramezanpour Z, Pourafrasyabi M, Ramezanpour Z (2014) Phytoplankton as bio-indicator of water quality in Sefid Rud River – Iran (South Caspian Sea). Casp J Environ Sci 12:31–40
Reddy GPO (2018) Satellite remote sensing sensors: principles and applications. In: Singh SK (ed) Reddy GPO. Springer International Publishing, Cham, pp 21–43
Resh VH (2007) Multinational, freshwater biomonitoring programs in the developing world: lessons learned from African and Southeast Asian River surveys. Environ Manag 39:737–748. https://doi.org/10.1007/s00267-006-0151-8
Respondek Z, Jerz D, Świsłowski P, Rajfur M (2022) Active biomonitoring of heavy metal concentrations in aquatic environment using mosses and algae. Water 14:3335. https://doi.org/10.3390/w14203335
Rivera SF, Vasselon V, Bouchez A, Rimet F (2020) Diatom metabarcoding applied to large scale monitoring networks: optimization of bioinformatics strategies using Mothur software. Ecol Indic 109:105775. https://doi.org/10.1016/j.ecolind.2019.105775
Rovira J, Domingo JL (2019) Human health risks due to exposure to inorganic and organic chemicals from textiles: a review. Environ Res 168:62–69. https://doi.org/10.1016/j.envres.2018.09.027
Ruppen D, Brugger F (2022) “I will sample until things get better – or until I die.” Potential and limits of citizen science to promote social accountability for environmental pollution. World Dev 157:105952. https://doi.org/10.1016/j.worlddev.2022.105952
Schwacke LH, Gulland FM, White S (2013) Sentinel species in oceans and human health. In: Laws EA (ed) environmental toxicology. Springer New York, New York, pp 503–528
Silva TT, Medeiros G, Amaral MWW et al (2022) Taxonomic and morphofunctional phytoplankton response to environmental variability in rivers from different hydrographic basins in Southern Brazil. Acta Limnol Bras 34:e23. https://doi.org/10.1590/S2179-975X1222
Simionov IA, Cristea DS, Petrea SM et al (2021) Preliminary investigation of lower Danube pollution caused by potentially toxic metals. Chemosphere 264:128496. https://doi.org/10.1016/j.chemosphere.2020.128496
Singh S, Kumar V, Dhanjal DS et al (2020) Biological biosensors for monitoring and diagnosis. In: Singh J, Vyas A, Wang S, Prasad R (eds) Microbial biotechnology: basic research and applications. Environmental and Microbial Biotechnology. Springer, Singapore, pp 317–335. https://doi.org/10.1007/978-981-15-2817-0_14
Sirunda J, Oberholster P, Wolfaardt G et al (2021) The assessment of phytoplankton dynamics in two reservoirs in Southern Africa with special reference to water abstraction for inter-basin transfers and potable water production. Water 13:3045. https://doi.org/10.3390/w13213045
Smucker NJ, Kuhn A, Cruz-Quinones CJ et al (2018) Stable isotopes of algae and macroinvertebrates in streams respond to watershed urbanization, inform management goals, and indicate food web relationships. Ecol Indic 90:295–304. https://doi.org/10.1016/j.ecolind.2018.03.024
Solak CN, Peszek Ł, Yilmaz E et al (2020) Use of diatoms in monitoring the Sakarya River basin, Turkey. Water (switzerland) 12:1–20. https://doi.org/10.3390/w12030703
Stat M, Huggett MJ, Bernasconi R et al (2017) Ecosystem biomonitoring with eDNA: metabarcoding across the tree of life in a tropical marine environment. Sci Rep 7:12240. https://doi.org/10.1038/s41598-017-12501-5
Steward AL, Datry T, Langhans SD (2022) The terrestrial and semi-aquatic invertebrates of intermittent rivers and ephemeral streams. Biol Rev 97:1408–1425. https://doi.org/10.1111/brv.12848
Sumudumali RGI, Jayawardana JMCK (2021) A review of biological monitoring of aquatic ecosystems approaches: with special reference to macroinvertebrates and pesticide pollution. Environ Manag 67:263–276. https://doi.org/10.1007/s00267-020-01423-0
Syeed MMM, Hossain MS, Karim MR et al (2023) Surface water quality profiling using the water quality index, pollution index and statistical methods: a critical review. Environ Sustain Indic 18:100247. https://doi.org/10.1016/j.indic.2023.100247
Talbot CJ, Bennett EM, Cassell K et al (2018) The impact of flooding on aquatic ecosystem services. Biogeochemistry 141:439–461. https://doi.org/10.1007/s10533-018-0449-7
Tapia PM (2008) Diatoms as bioindicators of pollution in the Mantaro River, Central Andes. Peru Int J Environ Heal 2:82. https://doi.org/10.1504/IJENVH.2008.018674
Thompson PA, Carstensen J (2023) Global observing for phytoplankton? A perspective. J Plankton Res 45:221–234. https://doi.org/10.1093/plankt/fbab090
Thornhill I, Ho JG, Zhang Y et al (2017) Prioritising local action for water quality improvement using citizen science; a study across three major metropolitan areas of China. Sci Total Environ 584–585:1268–1281. https://doi.org/10.1016/j.scitotenv.2017.01.200
Tolotti R, Consani S, Carbone C et al (2019) Benthic diatom community response to metal contamination from an abandoned Cu mine: case study of the Gromolo Torrent (Italy). J Environ Sci (china) 75:233–246. https://doi.org/10.1016/j.jes.2018.03.034
Torrisi M, Scuri S, Dell’Uomo A, Cocchioni M (2010) Comparative monitoring by means of diatoms, macroinvertebrates and chemical parameters of an Apennine watercourse of central Italy: the river Tenna. Ecol Indic 10:910–913. https://doi.org/10.1016/j.ecolind.2010.01.010
Tyovenda AA, Ikpughul SI, Sombo T (2019) Assessment of heavy metal pollution of water, sediments and algae in river Benue at Jimeta-Yola, Adamawa State, Nigeria. Niger Ann Pure Appl Sci 1:186–195. https://doi.org/10.46912/napas.44
Ugbeyide JA, Ugwumba OA (2021) Water quality and phytoplankton as indicators of pollution in Ibuya River. Br J Environ Sci 9:26–39
Vetrivel SA, Diptanghu M, Ebhin MR et al (2017) Green algae of the genus Spirogyra: a potential absorbent for heavy metal from coal mine water. Remediation 27:81–90. https://doi.org/10.1002/rem.21522
Wijngaard RR, van der Perk M, van der Grift B et al (2017) The impact of climate change on metal transport in a lowland catchment. Water Air Soil Pollut 228:107. https://doi.org/10.1007/s11270-017-3261-4
Wohl E (2018) Rivers as ecosystems. In: Wohl E (ed) Sustaining river ecosystems and water resources. SpringerBriefs in environmental science. Springer International Publishing, Cham, pp 11–58. https://doi.org/10.1007/978-3-319-65124-8_2
Wright K, Golder S, Rodriguez-Lopez R (2014) Citation searching: a systematic review case study of multiple risk behaviour interventions. BMC Med Res Methodol 14:73. https://doi.org/10.1186/1471-2288-14-73
Wu B, Ga L, Wang Y, Ai J (2023) Recent advances in the application of bionanosensors for the analysis of heavy metals in aquatic environments. Molecules 29:34. https://doi.org/10.3390/molecules29010034
Xia XH, Wu Q, Mou XL, Lai YJ (2015) Potential impacts of climate change on the water quality of different water bodies. J Environ Inf 25:85–98. https://doi.org/10.3808/jei.201400263
Yabe J, Ishizuka M, Umemura T (2010) Current levels of heavy metal pollution in Africa. J Vet Med Sci 72:1257–1263. https://doi.org/10.1292/jvms.10-0058
Yahya AN, Mohamed SK, Mohamed AG (2018) Environmental pollution by heavy metals in the aquatic ecosystems of Egypt. Open Access J Toxicol 3:555603. https://doi.org/10.19080/OAJT.2018.03.555603
Yilmaz N, Ozyigit II, Demir HH, Yalcin IE (2021) Assessment on phytoplankton composition and heavy metal pollution in a drinking water resource: Lake Terkos (Istanbul, Turkey). Desalin Water Treat 225:265–274. https://doi.org/10.5004/dwt.2021.27221
Yu H, Kong B, He Z-W et al (2020) The potential of integrating landscape, geochemical and economical indices to analyze watershed ecological environment. J Hydrol 583:124298. https://doi.org/10.1016/j.jhydrol.2019.124298
Zhou Q, Zhang J, Fu J et al (2008) Biomonitoring: an appealing tool for assessment of metal pollution in the aquatic ecosystem. Anal Chim Acta 606:135–150. https://doi.org/10.1016/j.aca.2007.11.018
Zhou Q, Yang N, Li Y et al (2020) Total concentrations and sources of heavy metal pollution in global river and lake water bodies from 1972 to 2017. Glob Ecol Conserv 22:e00925. https://doi.org/10.1016/j.gecco.2020.e00925
Zhu M, Wang J, Yang X et al (2022) A review of the application of machine learning in water quality evaluation. Eco-Environ Heal 1:107–116. https://doi.org/10.1016/j.eehl.2022.06.001
Download references
Acknowledgements
This research was supported by the Chair-Environment and Development, Oliver R. Tambo of the Africa Research Initiative (ORTARChI) Project at the Copperbelt University, Zambia. ORTARChI is an initiative of South Africa’s National Research Foundation (NRF) and the Department of Science and Innovation (DSI) in partnership with the Oliver and Adelaide Tambo Foundation (OATF), Canada’s International Development Research Centre (IDRC), and the National Science and Technology Council (NSTC) of Zambia. The findings and conclusions in this publication are those of the authors and should not be construed to represent any official position of the organisations that funded the study.
Open access funding provided by University of Pretoria. This review was funded by the Chair-Environment and Development, Oliver R. Tambo of the Africa Research Chair Initiative (ORTARChI), Copperbelt University, Zambia.
Author information
Authors and affiliations.
Department of Biological Sciences, School of Mathematics & Natural Sciences, Copperbelt University, P. O. Box 21692, Kitwe, Zambia
Mary Mulenga & Todd Johnson
Department of Zoology & Aquatic Sciences, School of Natural Resources, Copperbelt University, P. O. Box 21692, Kitwe, Zambia
Concillia Monde & Kennedy O. Ouma
Department of Plant & Environmental Sciences, School of Natural Resources, Copperbelt University, P. O. Box 21692, Kitwe, Zambia
Stephen Syampungani
Chair-Environment & Development, Oliver R Tambo Africa Research Chair Initiative (ORTARChI), Copperbelt University, P. O. Box 21692, Kitwe, Zambia
Mary Mulenga, Concillia Monde & Stephen Syampungani
Forest Science Postgraduate Program, Department of Plant & Soil Sciences, Plant Sciences Complex, University of Pretoria, Private Bag x20, Hatfield, Pretoria, 0002, South Africa
You can also search for this author in PubMed Google Scholar
Contributions
Conceptualization, M.M., C.M., and K.O.; methodology, M.M., K.O., and S.S; validation, M.M., C.M., K.O., T.J., and S.S.; formal analysis, M.M., C.M., and K.O.; investigation, M.M and K.O.; literature and data curation, M.M. and K.O.; writing—original draft, M.M., C.M., K.O., T.J., and S.S.; writing—review and editing, M.M., C.M., K.O, T.J., and S.S.; supervision, C.M., T.J., and S.S. All authors have read and agreed to the published version of the manuscript.
Corresponding author
Correspondence to Mary Mulenga .
Ethics declarations
Competing interests.
The authors declare no competing interests.
Additional information
Responsible Editor: Robert Duran
Publisher's Note
Springer Nature remains neutral with regard to jurisdictional claims in published maps and institutional affiliations.
Rights and permissions
Open Access This article is licensed under a Creative Commons Attribution 4.0 International License, which permits use, sharing, adaptation, distribution and reproduction in any medium or format, as long as you give appropriate credit to the original author(s) and the source, provide a link to the Creative Commons licence, and indicate if changes were made. The images or other third party material in this article are included in the article's Creative Commons licence, unless indicated otherwise in a credit line to the material. If material is not included in the article's Creative Commons licence and your intended use is not permitted by statutory regulation or exceeds the permitted use, you will need to obtain permission directly from the copyright holder. To view a copy of this licence, visit http://creativecommons.org/licenses/by/4.0/ .
Reprints and permissions
About this article
Mulenga, M., Monde, C., Johnson, T. et al. Advances in the integration of microalgal communities for biomonitoring of metal pollution in aquatic ecosystems of sub-Saharan Africa. Environ Sci Pollut Res (2024). https://doi.org/10.1007/s11356-024-33781-1
Download citation
Received : 06 December 2023
Accepted : 16 May 2024
Published : 01 June 2024
DOI : https://doi.org/10.1007/s11356-024-33781-1
Share this article
Anyone you share the following link with will be able to read this content:
Sorry, a shareable link is not currently available for this article.
Provided by the Springer Nature SharedIt content-sharing initiative
- Bioindicator
- Microalgal e-DNA
- Biomonitoring
- Artificial intelligence
- Find a journal
- Publish with us
- Track your research

Health & Environmental Research Online (HERO)
- Learn about HERO
- Search HERO
- Projects in HERO
- Risk Assessment
- Transparency & Integrity

An official website of the United States government
Here’s how you know
Official websites use .gov A .gov website belongs to an official government organization in the United States.
Secure .gov websites use HTTPS A lock ( Lock A locked padlock ) or https:// means you’ve safely connected to the .gov website. Share sensitive information only on official, secure websites.
JavaScript appears to be disabled on this computer. Please click here to see any active alerts .
Celebrating EPA Researcher
EPA environmental epidemiologist Shannon Griffin received the 2023 Arthur S. Flemming Award, which honors outstanding federal employees, for creatively advancing laboratory-based biomarker techniques.
Read more here
Healthy & Resilient Communities Research Webinar
Join us at our June 11th webinar from 3:00 -4:00 p.m. ET to hear from an EPA researcher about geospatial model development and applications in cumulative impacts research.
Register here
Science is the foundation
EPA is one of the world’s leading environmental and human health research organizations.
The Office of Research and Development is EPA's scientific research arm. On this page you can access our products, tools, and events, and learn about grant and job opportunities.
Research Topics
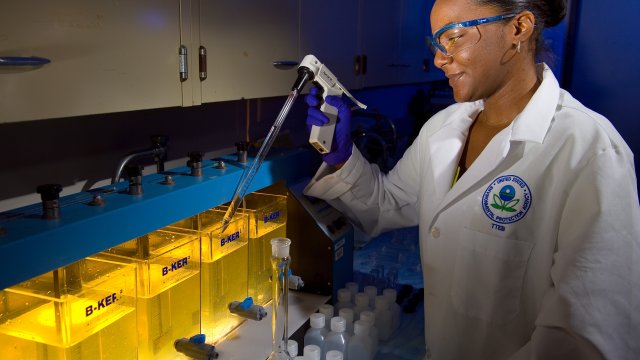
EPA does research on many different topics, including air, water, climate, and more.
Publications
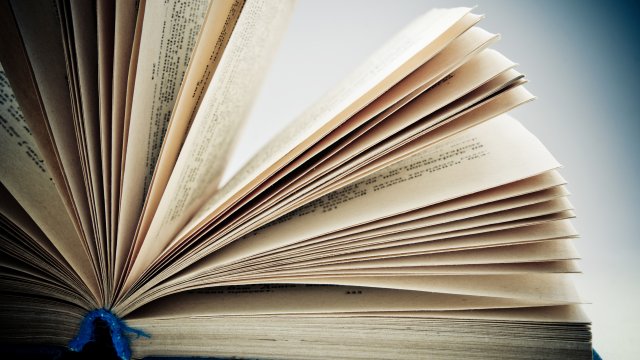
Read the latest scientific publications, reports, articles, and more.
Funding & Career Opportunities
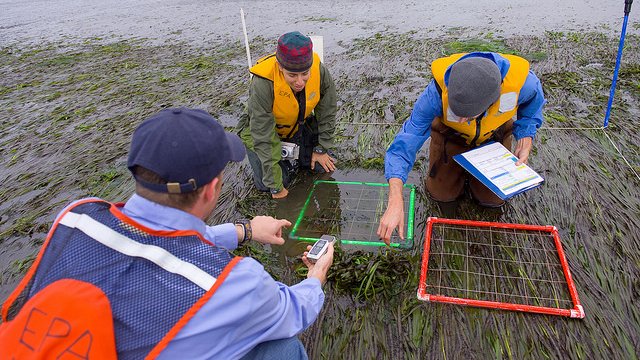
Find research funding opportunities, small business grants, jobs, and ways to license EPA’s technology.
Upcoming Events
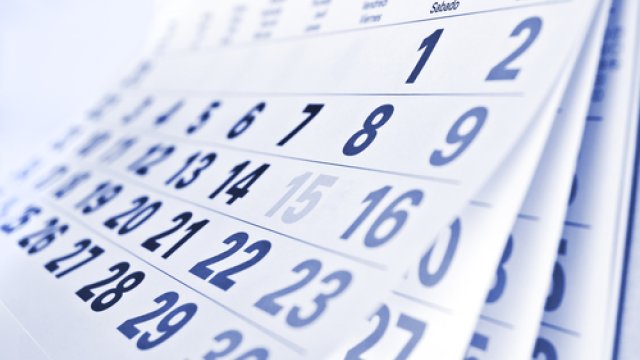
See what webinars and events EPA researchers are hosting, presenting at, or attending.
Stay Connected
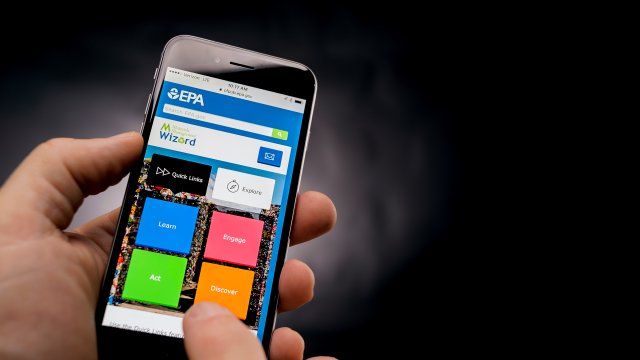
Keep up to date with the latest Science Matters newsletter, social media posts, and press releases.
About Our Research
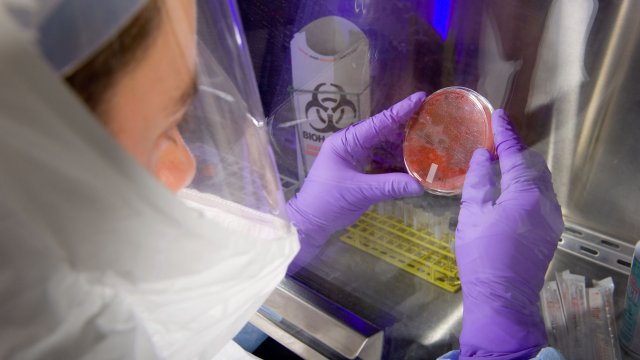
Learn about EPA’s research organization, research facilities, and the research planning process.
Research Tools
EPA Science Models and Research Tools (SMaRT) Search is a searchable inventory of freely available models, tools, and databases from EPA's Office of Research and Development (ORD).
In Your Community
See how EPA's Office of Research and Development (ORD) engages and collaborates with states , including tools and other resources available to states.

Environmental Science: Water Research & Technology
Urea and ammonium fluoride di-nitrogen and cu & fe bi-metal co-doped carbon felt as cathode for electro-fenton degradation of norfloxacin: 1 o 2 -dominated oxidation pathway †.

* Corresponding authors
a Department of Environmental Engineering, Taiyuan University of Technology, Jinzhong 030600, Shanxi Province, China E-mail: [email protected].
b Shanxi Key Laboratory of Compound Air Pollutions Identification and Control, Taiyuan University of Technology, Jinzhong 030600, Shanxi Province, China
c Department of Biological and Pharmaceutical Engineering, College of Biomedical Engineering, Taiyuan University of Technology, Jinzhong 030600, Shanxi Province, China
Carbon materials co-doped with both metals and non-metallic heteroatoms have become an important research focus as catalysts for heterogeneous electro-Fenton technology and the removal of refractory organics. However, there is still a lack of in-depth studies on the doping of carbon with multiple nitrogen sources and their possible synergistic mechanism. In this study, two types of nitrogens (urea and ammonium fluoride) and Cu&Fe bimetal co-doped carbon felt electrodes (C–CuFe/N) were designed to explore the effect of co-doping on carbon. C–CuFe/N exhibited a high degradation efficiency towards norfloxacin (97.2%), low ion leaching and high cycling stability (90.3% after ten cycles), better than those shown by C–CuFe, C–CuFe/UN and C–CuFe/FN prepared with none or a single nitrogen dopant. Various characterizations indicated that C–CuFe/N has the largest specific surface area, highest content of Fe( II ), most surface oxygen and active sites, and importantly, stable M–N x bond, indicating that UN and FN exhibited an excellent synergistic effect. According to the quenching experiments, the dominant reactive oxygen species for C–CuFe, C–CuFe/UN and C–CuFe/FN were radical species (·OH and O 2 ˙ − ), while they changed to non-radical species ( 1 O 2 ) for C–CuFe/N under acidic condition. Alternatively, C–CuFe/N showed a good catalytic performance (97.2–92.3%) over a wide initial pH range (1.2–11.3), but during the degradation process, all the pH values changed toward neutral, and the oxidation pathway varied from 1 O 2 -dominated under acidic condition to radical-dominated under neutral or alkaline condition. Generally, a good synergistic effect was found to exist between the dual nitrogens, which promoted the catalytic activity as well as stability of the catalyst, thus providing a good strategy to design catalysts.
Supplementary files
- Supplementary information PDF (1670K)
Article information
Download citation, permissions.

Urea and ammonium fluoride di-nitrogen and Cu & Fe bi-metal co-doped carbon felt as cathode for electro-Fenton degradation of norfloxacin: 1 O 2 -dominated oxidation pathway
J. Li, L. Gao, Y. Chen, X. Meng, X. Li, K. Qi and J. Zhang, Environ. Sci.: Water Res. Technol. , 2024, Advance Article , DOI: 10.1039/D4EW00210E
To request permission to reproduce material from this article, please go to the Copyright Clearance Center request page .
If you are an author contributing to an RSC publication, you do not need to request permission provided correct acknowledgement is given.
If you are the author of this article, you do not need to request permission to reproduce figures and diagrams provided correct acknowledgement is given. If you want to reproduce the whole article in a third-party publication (excluding your thesis/dissertation for which permission is not required) please go to the Copyright Clearance Center request page .
Read more about how to correctly acknowledge RSC content .
Social activity
Search articles by author.
This article has not yet been cited.
Delivering hydrogen to EU’s industry: which are the greenest options?
Using hydrogen produced from abundantly available renewables on-site is the most sustainable option. Delivering compressed renewable hydrogen via pipelines or shipping liquid renewable hydrogen could still be environmentally friendly, research says.
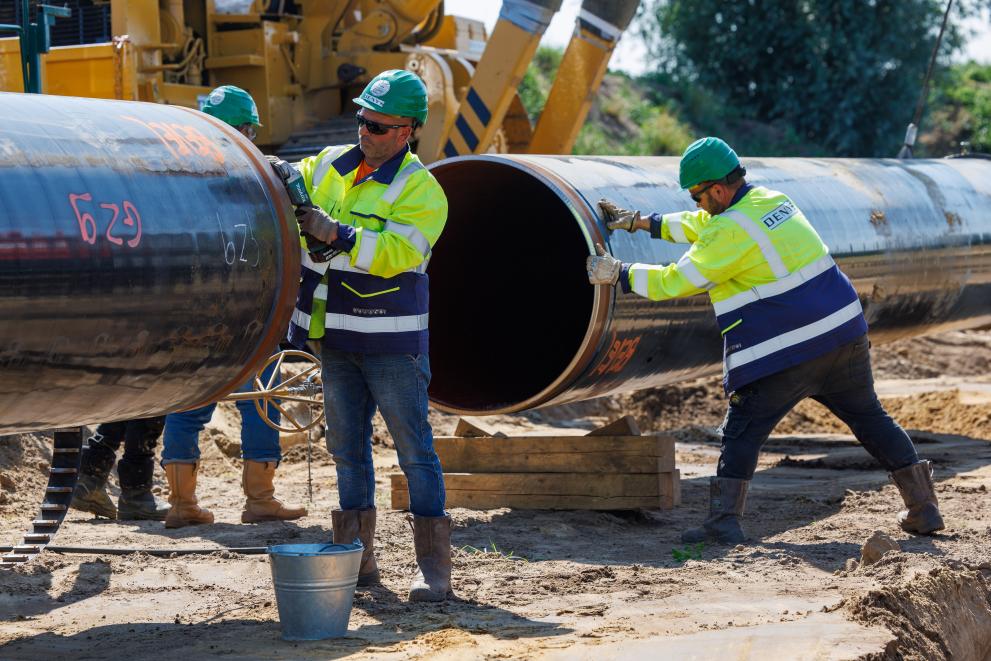
Renewable hydrogen is expected to play a crucial role in reducing carbon emissions in Europe. Previous JRC research revealed that sourcing it from regions with cheaper renewable energy can prove to be more cost-effective than local production.
However, environmental concerns arise from transporting large quantities of hydrogen over long distances, as the environmental impact varies significantly according to the production technology and the method of delivery.
To address these concerns, a new study compares the life cycle environmental impacts of on-site production through steam methane reforming (SMR) or electrolysis with three different delivery methods, including compression, liquefaction, and chemical bonding to other molecules. Transportation by both ship and pipeline was considered.
The distance used to compare the different methods of delivery is 2,500 km, compatible with the extent of EU territory and equivalent to the distance between Portugal and the Netherlands. The two countries were considered based on a proposal in an EU funded project which examined the feasibility of sustainable hydrogen transportation.
The results show that the environmental performance of hydrogen supplied to large industries can vary significantly based on the production technology and delivery pathway.
The study was carried out by the JRC for the Clean Hydrogen Partnership , a public-private partnership supporting research and innovation (R&I) activities in hydrogen technologies in Europe. The findings result in key recommendations for policymakers and stakeholders to help countries and industries to accelerate the transition towards a more sustainable hydrogen economy.
On-site production versus long-distance delivery
The most environmentally sustainable approach is on-site production using efficient renewable sources, such as wind power in the Netherlands. If on-site production is not viable using local abundant renewable sources, importing renewable hydrogen can still lead to a significant reduction in greenhouse gas (GHG) emissions compared to on-site production with fossil fuels. However, focusing solely on GHG emissions may lead to other, unintended environmental impacts.
Shipping liquid hydrogen and transporting compressed hydrogen through pipelines appear to have the least environmental impact when delivering hydrogen over long distances.
Meanwhile, the process of packing and unpacking hydrogen into chemical carriers such as ammonia, liquid organic compounds, methanol, and synthetic natural gas demands larger amounts of energy and resources. It makes these options less desirable to minimise environmental impact. But no significant difference was noticed in comparative environmental impact of delivery methods when comparing chemical carriers one with another.
Role of renewable energy infrastructure
The report emphasises the close relationship between the environmental impact of delivered hydrogen and renewable energy infrastructure.
For imported solar-generated hydrogen to have an environmental advantage over conventional hydrogen production from fossil fuels, the environmental impact of generating electricity through photovoltaic panels must be significantly reduced.
This can be achieved by improving the efficiency of photovoltaic panels in terms of materials use and utilising renewable energy for their production.
Impact of water use
Water use is another crucial factor to consider. The availability of freshwater affects the impact of hydrogen production. On-site hydrogen generation in water-rich countries proves to be a more sustainable option in terms of water use compared to importing hydrogen from water-scarce nations.
Hydrogen loss
Hydrogen losses during the delivery chain can significantly increase the environmental impact of delivered hydrogen. However, options that are more susceptible to losses, such as liquid and compressed hydrogen, still have lower environmental impacts than using hydrogen carriers.
When on-site production of hydrogen using local renewable sources is not feasible, importing renewable hydrogen from closer regions becomes the more environmentally sustainable choice. When transporting hydrogen over long distances within Europe, delivering compressed hydrogen through pipelines or liquid hydrogen via ships stands out as the preferred option in terms of environmental impact.
Related links
Environmental life cycle assessment (LCA) comparison of hydrogen delivery options within Europe
Clean Hydrogen Partnership
EU Hydrogen strategy
More news on a similar topic
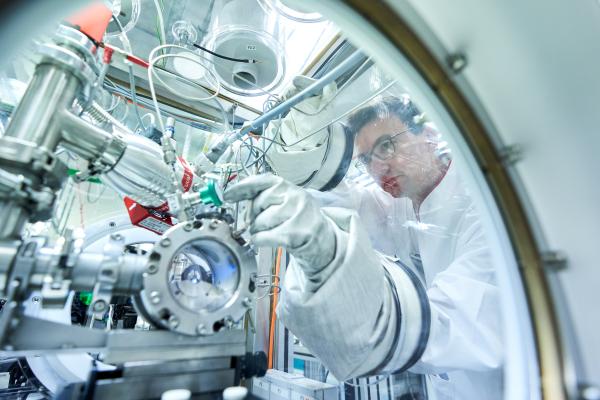
- General publications
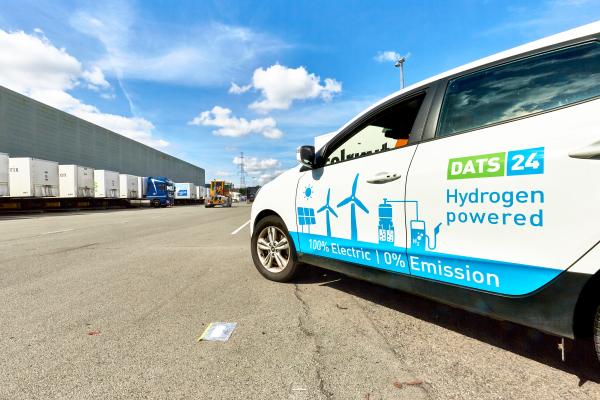
- 6 March 2024
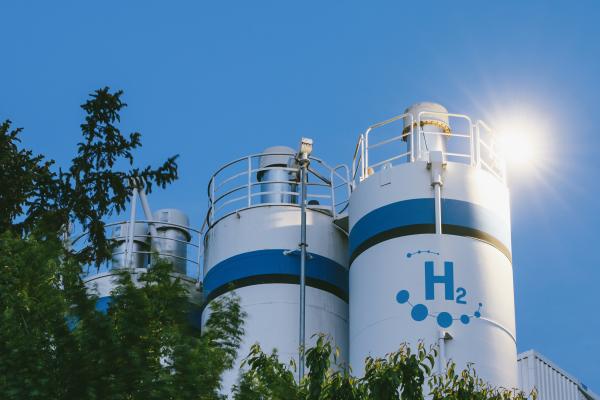
- 24 November 2023
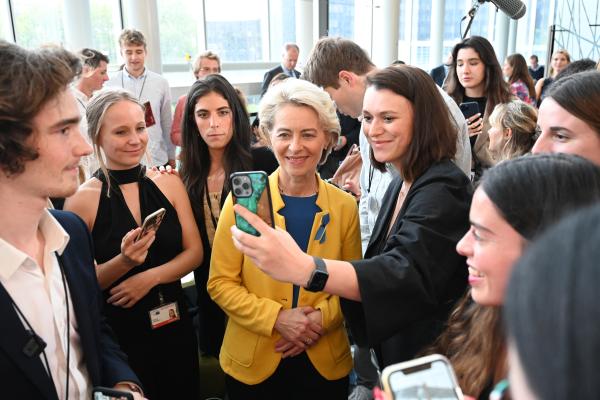
- News announcement
- 11 September 2023
- 10 min read
Share this page

IMAGES
VIDEO
COMMENTS
Published since 1928, Water Environment Research (WER) is an international multidisciplinary water and wastewater research journal. We focus on the dissemination of fundamental and applied research in all scientific and technical areas related to water quality. We encourage communication and interdisciplinary research between water sciences and ...
Water Environment Research (WER) is a peer-reviewed journal that publishes research on water quality and resource recovery since 1928. It covers topics such as administration, biological treatment, environmental toxicology, groundwater, reclamation, and more.
Published since 1928, Water Environment Research (WER) is an international multidisciplinary water resource management journal for the dissemination of fundamental and applied research in all scientific and technical areas related to water quality and resource recovery.WER's goal is to foster communication and interdisciplinary research between water sciences and related fields such as ...
Water is a vital resource for human society and the natural environment, and water-related research has been the subject of increasing attention from the scientific community and policymakers in recent years. 12 A bibliometric analysis of water-related research published in the Scopus database during the period of 2018-22 was conducted to explore the research trends, patterns, and gaps in ...
Nature Water is a monthly online journal publishing research on the evolving relationship between water and society, engineering, the environment and SDGs ...
Zhuang, W. Eco-environmental impact of inter-basin water transfer projects: a review. Environ. Sci. Pollut. ... Center for Water Resources Research, School of Agricultural, Earth and Environmental ...
A journal that showcases high quality research about sustainable water, covering treatment, distribution, management, and policy. It publishes original articles, reviews, perspectives, and comments on various topics related to engineered water systems and the built environment.
A peer-reviewed journal that publishes original research and reviews on water-related topics. Find articles on innovation, sustainability, water quality, treatment, and more.
Water Environment Research is a multidisciplinary journal on water quality and resource recovery. It covers topics such as ecological modeling, environmental chemistry, pollution, waste management, and water science and technology. See its scope, publication type, ISSN, and SJR quartiles.
Water Research publishes original research papers on all aspects of the science and technology of the anthropogenic water cycle, water quality, and its management worldwide. The journal covers topics such as treatment processes, urban hydrology, drinking water, reuse, sanitation, contaminants, environmental restoration, and modelling.
The breathtaking economic development put a heavy toll on ecology, especially on water pollution. Efficient water resource management has a long-term influence on the sustainable development of the economy and society. Economic development and ecology preservation are tangled together, and the growth of one is not possible without the other. Deep learning (DL) is ubiquitous in autonomous ...
Diversity in Research Jobs Find your next job in healthcare, the sciences and academia. Books. Search by Subject Browse our catalog of books. ... Water Environment Research. Download Product Flyer; Permissions; Water Environment Research. Edited By:Jason He. Print ISSN: 1061-4303 Online ISSN: 1554-7531.
Research to Protect Our Water Resources. As changing climate patterns, biological and chemical contaminants, and aging water infrastructure systems threaten the availability and quality of water, communities and aquatic ecosystems will increasingly rely on advances in science and technology for resilience.
The Water Research Foundation (WRF) is the leading research organization advancing the science of all water to meet the evolving needs of its subscribers and the water sector. ... (Denver, CO) 02/6/23 - The Water Research Foundation and Water Environment Federation are pleased to invite teams to participate in the fifth annual Intelligent ...
AI and Big Data in Water Environments. This collection of papers published in ACS ES&T Water look at the current progress, research, opportunities and challenges in applying artificial intelligence, machine learning and data analytics to solving environmental problems related to water. Read the Virtual Issue.
Another use of a significant amount of water is to support the natural environment along the river, including plants and wetlands. ... (2024, May 29). Research to uncover the impact of water use ...
The Water Environment Research Foundation and the WateReuse Research Foundation have merged to form the Water Environment & Reuse Foundation (WE&RF; Alexandria, Va.). The merger, announced May 9, brings together the organizations' expansive portfolio of research on water, wastewater, and stormwater topics. It also reflects on the sector's movement toward the concept of "one water," […]
Overview. Aims and Scope: Published since 1928, Water Environment Research (WER) is an international multidisciplinary water resource management journal for the dissemination of fundamental and applied research in all scientific and technical areas related to water quality and resource recovery.WER's goal is to foster communication and interdisciplinary research between water sciences and ...
Stream ecosystems play an ecologically significant role in supporting aquatic biodiversity and providing beneficial ecosystem services that sustain the environment and promote human well-being (Limburg 2009; Maes et al. 2020).Stream ecosystem services include fresh water and food provisioning, sediment retention and transport, pollution control, recreation and ecotourism, flood regulation ...
Today, the White House Office of Science, Technology, and Policy (OSTP) released the "National Strategy for Aquatic Environmental DNA.". The Environmental DNA (eDNA) Task Team had 10 federal agencies participating in drafting the strategy and included significant scoping and engagement of the private sector, academia and non-profit ...
United States Environmental Protection Agency. Search Search. Main menu. Environmental Topics; Laws & Regulations; About EPA; Health & Environmental Research Online (HERO) ... Water Resources Research ISSN: 0043-1397 EISSN: 1944-7973 Volume. 49 Issue. 3 Page Numbers. 1483-1502 DOI. 10.1002/wrcr.20147 Web of Science Id. WOS ...
Science is the foundation. EPA is one of the world's leading environmental and human health research organizations. The Office of Research and Development is EPA's scientific research arm. On this page you can access our products, tools, and events, and learn about grant and job opportunities.
Carbon materials co-doped with both metals and non-metallic heteroatoms have become an important research focus as catalysts for heterogeneous electro-Fenton technology and the removal of refractory organics. However, there is still a lack of in-depth studies on the doping of carbon with multiple nitrogen sources a ... Environmental Science ...
Published since 1928, Water Environment Research (WER) is an international multidisciplinary water and wastewater research journal. We focus on the dissemination of fundamental and applied research in all scientific and technical areas related to water quality. We encourage communication and interdisciplinary research between water sciences and ...
Renewable hydrogen is expected to play a crucial role in reducing carbon emissions in Europe. Previous JRC research revealed that sourcing it from regions with cheaper renewable energy can prove to be more cost-effective than local production.. However, environmental concerns arise from transporting large quantities of hydrogen over long distances, as the environmental impact varies ...