An official website of the United States government
Official websites use .gov A .gov website belongs to an official government organization in the United States.
Secure .gov websites use HTTPS A lock ( Lock Locked padlock icon ) or https:// means you've safely connected to the .gov website. Share sensitive information only on official, secure websites.
- Publications
- Account settings
- Advanced Search
- Journal List

A review on prospective production of biofuel from microalgae
Ramya ganesan, s manigandan, melvin s samuel, rajasree shanmuganathan, kathirvel brindhadevi, nguyen thuy lan chi, pham anh duc, arivalagan pugazhendhi.
- Author information
- Article notes
- Copyright and License information
Corresponding author. [email protected]
Received 2020 May 5; Revised 2020 Jul 15; Accepted 2020 Jul 21; Collection date 2020 Sep.
This is an open access article under the CC BY-NC-ND license (http://creativecommons.org/licenses/by-nc-nd/4.0/).
Graphical abstract
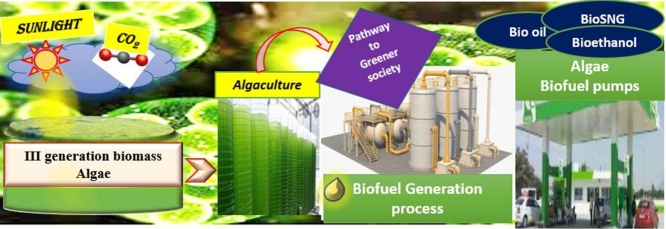
Keywords: Biofuel, Microalgae, III generation fuels, Cultivation, Pyrolysis
Challenges faced in I generation biofuels and transition to II generation biofuels.
Microalgae as a feedstock for biofuel production has been discussed in this review.
Two stage cultivation strategies and extraction techniques were discussed.
HTL, fermentation, transesterification and pyrolysis of III generation biomass in briefly analyzed.
This critical review summarizes the utilization of algae as the resilient source for biofuel. The paper validates the different stages in generation of biofuels and provides a clarity on III generation biofuels. The microalgae is focused as an incredible source and a detailed discussion has been carried out from the cultivation, extraction and conversion to the final product. An elaborate view on conversion methodologies and troubles involved in the respective techniques are presented. The efficiency of the algal fuel performing in I/C engines derived from major techniques is considered. There exist new challenging barriers in the implementation of microalgae as prospective source in the energy market. In addition, types of pyrolysis for the production of main product from microalgae had been discussed in detail. Besides, some microalgae grow easily from fresh to waste water, make it more feasible source. Although the microalgae are a best alternative, cost of production and the yield of biofuel are still challenging. Further, cultivation of microalgae is very effective by applying two stage cultivation strategies. This comprehensive review provides the useful tool to identify, innovate and operate microalgae as the potential based biofuel.
1. Introduction
Over the past few decades the world has witnessed the energy thirst and exploitation of fuels by developing countries mainly India and Africa to satisfy the raising standards [ 1 ]. The major concerns associated with the existing energy insecurity are rapid industrialization consuming fossil fuels, record high gasoline process, scaling the dependency on middle east oil sources, negative impact of fossil resources on greenhouse gas emissions forcing the pressure on society, increased levels of NOX, SOX and metal particles in atmosphere due to the usage of present day fuels [ 2 , 3 ]. Beginning from the individual country’s thirst, changes in environmental scenario and friction between nations with respect to fuel consumption, the drives are challenging and hence has to be answered. Once, the world’s energy search was indolent without the track of source. But, today in 20th century it is really indeed close to loop with the aid of propitious choices in the direction of biofuels. The endless expectations of the society for a fuel which can be the savior of present day problems points at limited sources. Within a decade span, developing countries with the large interest towards economy growth have consumed 2/3rd of energy than previous years ( Fig. 1 ). International Energy Agency (IEA) has summarized the global energy demand to be faced in near future along with and Organization for economic co-operation and development (OECD). According to late economist Angus Maddison, the developing countries would surpass the developed countries by 2030 in economic weight. India and China have all the key sources for the improvement of the poor country involving investment and trading [ 4 ]. “South – South links” is important sources for development. Though this has improved the overall world economy, significant decline in reserves is also a known fact.
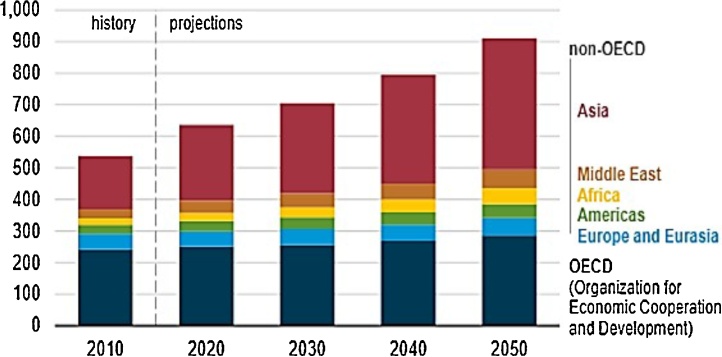
Energy consumption by Region. [ 5 ] Source: https://www.eia.gov/todayinenergy/detail.php?id=41433 .
The global oil need is constrained with limited number of countries compared to the large energy seeking countries. Treasures of 260 billion barrels and 115 billion barrels of oil makes Saudi Arabia as the king in the market followed by Iraq [ 6 ]. For energy security reasons, it is important to search for other oil suppliers including Nigeria and Angola where the oil production may rise to nearly 10 million barrels/ day by 2030.With the sense of urgency and insecurity in energy supply, China is receiving its load from more than 20 countries around the world. Initiatives have been taken by Putin’s government to choose route of pacific coast to Siberia field for Russia’s energy demand. This shows globally the hunt for new sources is also a key concern for the search of novel fuel. The Earth Summit held by UN conference in Rio de Janeiro during 3rd to 14th June in 1992 paved the path for environmental concerns including greenhouse effect and acid rain due to the GHG (Greenhouse gas) emissions. Kyoto protocol, a committee of Europe, Japan and Russia aims at CO 2 reduction levels [ 7 ]. Developing countries on the surge to industrialization rely on the large quantity of coal and other fossils which results in enhanced release of CO 2 gas.
Petro- fuels satisfies almost 94% of energy needs in road transportation division. Almost nine countries accounts for consumption of 75% liquid fuels and interestingly these are developing landscapes ( Fig. 2 ).
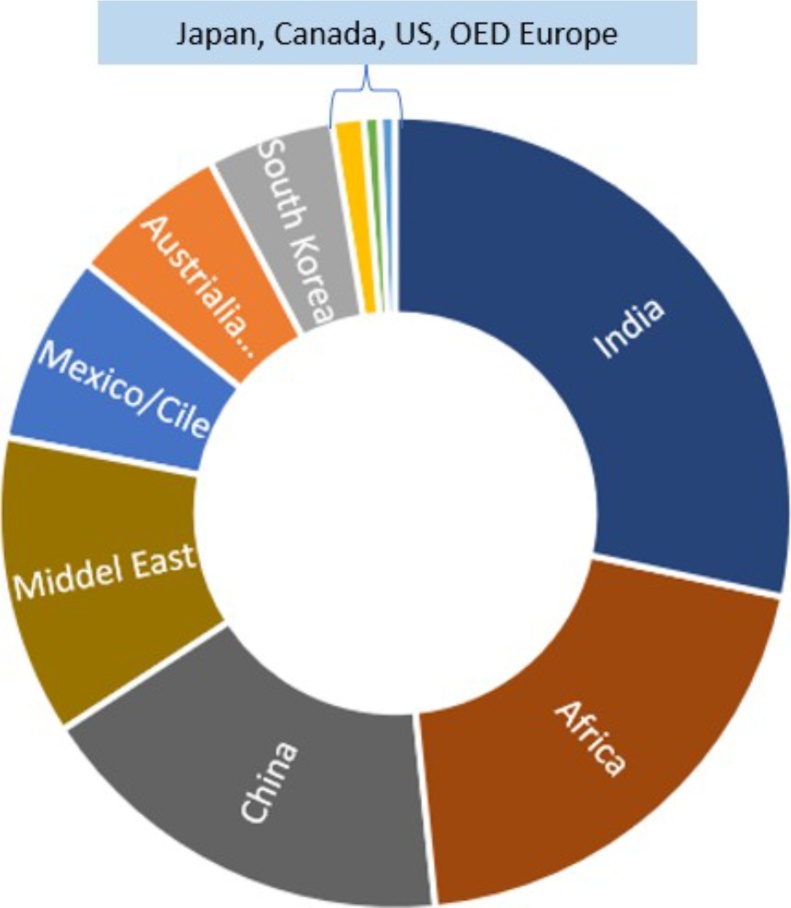
Country wise consumption of road transportation fuels.
The perpetual increase in CO 2 will definitely end with significant global warming effect. The prediction indicated that by 2030, the levels of CO 2 may almost triple by the spurring motor vehicle population. These critical issues poses strong emphasizes on the present generations fuel and also drives us towards the new alternative fuel ( Fig. 3 ). Research is pioneered in replicating this type of liquid fuels from renewable sources and surprisingly biomass is the only source that yields all the 3 phases of fuel. This review features the diverse plants, seeds and crops in the league of biofuel and classified as generations of biofuel. The stages of biofuel has raised from 1st generation to 3rd generation which decides the future of energy harnessing. The 1st generation biofuels is known for their potential of reducing CO 2 emissions while they are criticized a lot for the land usage and food shortage. The lignocellulosic biomass and waste animal oils constitute 2nd generation biofuels. The literature survey gives the difficulties with the 1st and 2nd generation biofuels. With the classy results in the production of bio-oils they can make a good choice but the problems faced with the techniques and cost ineffectiveness lags the chance in the race. The 3rd generation spots the viability of algae among the single celled organism (SCO) as an excellent alternative in fuel market. The article ventures the positive aspects of these microbodies with elaboration about the methods involved in growth and extraction of lipids from the oil rich species. Various techniques developed to convert the lipids or the whole algae into fuels and engine aspects of fuel derived from different methods are also discussed.
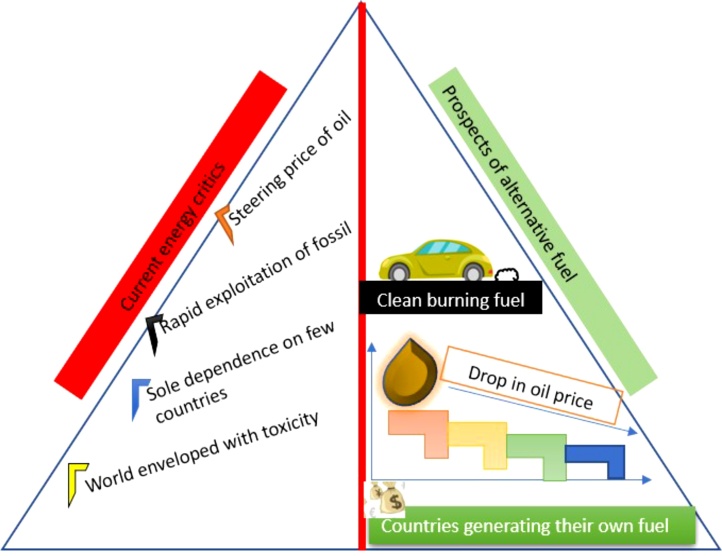
Present and future energy scenario.
2. Biofuels
Fossil fuels are formed beneath the earth’s crust over the period of years by the process called as fossilization. The fuel which is obtained from a chemical method from biomass rather than a slow geological process is known as Biofuel. U.S. Energy Information Administration (EIA) names the fuel and the same is followed in most of the countries. The liquid and gaseous form of fuels are generally termed as biofuels and have high utility in transportation sector. These fuels are easy to blend with the existing liquid fuels namely gasoline and diesel. The various forms of liquid biofuels are bio-alcohol, bio-diesel, bio-oil and group of gasoline, kerosene & diesel. The mixture of gasoline and alcohol derived from saccharification reactions is known as “Gasohol”. The gaseous biofuel is known as biogas and is rich in methane gas. Bio-alcohol may comprise bio methanol, bioethanol whereas bio-gas has major products. The biofuels are expected to satisfy at least one-fourth of the globe’s energy demand in the mid of 20th century. Three generations of biomass are under focus for the delivery of alternative fuels.
2.1. I generation (G1) biomass derived biofuels
In the debate between biofuels, it is necessary to distinguish the generation technologies as I and II generation. 1st generation biofuels comprises of both liquid and gaseous types of fuel. Biodiesel, vegetable oil, bio-ethers and bio-alcohol are the liquid forms while forms of biofuels. The I generation (G1) biomass are the oil bearing seeds and edible crops namely starch, sugarcane, animal fats, sunflower, rapeseed and palm. The complex triglycerides molecules with different alkyl chain lengths make each oil molecule unique. Triglycerides are three fatty acid units which are linked to an ester molecule and these triglycerides can be converted to biofuels with the type of methodology followed. The techniques used for the generation of fuels are transesterification, anerobic decomposition, fermentation and pyrolysis. The I generation biofuels wither can be used without blending or can be improvised by blending. Table 1 lists the utilization of edible (G1) crop oils such as mustard, rice bran, lemon seed, wheat germ, coconut, soyabean, rapeseed, sunflower, almond, walnut and pistachio in the biofuel production [ [8] , [9] , [10] , [11] , [12] , [13] , [14] , [15] , [16] , [17] , [18] ]. Europe, N. America, S. America and Asian countries function biodiesel plants with castor, sunflower, palm, and jatropha oil sources. Corn and sugarcane are considered to be fortune makers in India, US, France, China, Germany and Australia. According to Lowa State University, 2013 the expenditure of ethanol producing corn plant is nearly 0.75 US$ per litre annually which includes installation, feedstock availability, production and transportation cost. Despite of reduction GHG emissions and atmospheric pollution; they interfere with food security and social conflicts. Hence, with the incorporation of the views of stakeholders in ranking & evaluating the specific generation, it raises question on the sustainability of G1 fuels for the future.
Data of varieties of feedstocks for biofuels.
2.2. II generation biofuels (G2)
G2 fuels or advanced fuels are an assured alternative to GI fuels because of the source being non-food biomass. The by-products of food processing industry and wooden factories include inedible parts ex. dry wood, stalks of corn and wheat constitute 2nd generation biomass. Used oil products from restaurants, animal wastes and oil crops likely jojoba, jatropha and sea mango are also available materials as this next generation resource [ [19] , [20] , [21] , [22] , [23] , [24] , [25] , [26] , [27] , [28] ]. Types of nonedible converted to biofuels and the process used for production is given in Table 1 . The products caters from ethanol derived from cellulose to bio syngas (BioSNG); a mixture of hydrogen and carbon mono oxides with different technical approaches. Fermentation of saccharides, gasification of dried biomass, BtL (biomass to liquid) technology and HTL (hydrothermal liquification) of vegetable oils are the major process involved in G2. The gasification of dried biomass yields the lighter fuel biohydrogen, fermentation of cellulose & syngas delivers ethanol, butanol (C 4 H 9 OH) & methanol, FT synthesis followed by BtL provides C5-C18 hydrocarbon fuels. Comparatively, these fuels are in line with EU-RED (EU- Renewable Energy Directive), eco-friendly, clean burning, non-corrosive, does not lead to deforestation and also it is not used as fodder for animals due to its trace level of toxicity [ [29] , [30] , [31] , [32] ]. This perspective of advanced energy has accumulated investors around the world for the synthesis of biodiesel and bioethanol. Biodiesel using re used oils (cooking, tallow and vegetable oils) by Australian Renewable fuels Limited and bioethanol from wheat straws, agricultural residues, wood chips and sugarcane bagasse by BlueFire Ethanol Fuels, Inc, US, Cosan, Brazil and Coskata, US are to be mentioned. However, the outcome of G2 is still meagre compared to G1 with former producing five hundred million gallons while the latter outshines with fifteen billion gallons. In addition, the lack of proper technique and rich content of saturated fatty acid for biofuel production from the second generation feedstocks propels this choice as temporary. The search for the renewable and clean fuel has paved its path to III generation biofuels (G3).
2.3. A bloom in biofuel market – Algae – III generation biofuels (G3)
The bright or dark green patches found in wet regions are algae, the non-flowering plants like species containing chlorophyll yet distinct from floras ranging from micro to macro sizes. The processing of these microorganism diversifies the scope in nutritional industry, bioplastics, pharmaceuticals, special chemicals manufacture, organic fertilizer and the flourishing biofuel industry. The distinctive properties of algae such as : a) CO 2 absorbance for the growth helps in reduction of green-house effect, b) they do not require large area for development compared to other food crops, c) can adjust to brine water and d) also their lipid content is found high [ 33 , 34 ]. In early 19th century methane production from algae won a big momentum during energy crisis. Harder and Von Wiltsch proposed the algae as source of food and energy half a century ago. World’s first biodiesel plant suing algae proposed the algae as source of food and energy in mid 19th century. Japan, England and Israel began the cultivation of Chlorella algae on large scale during II world war. The abundance of fossil fuels diverted the idea of using these algae in Energy production to food commodities. The official Program “The aquatic species” was initiated with 25 million $ by US 18 years ago [ 34 ]. In recent years, the objective to be benefited by algae in synthesizing alternate fuel has been of great interest and they can significantly replace the G1 & G2 biomass. The lipids in algae can be converted to biodiesel by the generalized method used for conversion of vegetable oil into biodiesel. Whereas bioethanol and biobutanol are prepared from carbohydrates of algae. The market value of other biofuels is just half of the algal biofuel (420 US million $). This Fig. 3 would soar in the near future with proper technology practice. Few algae that are of research interest are Chlamydomonas reinhardtii (21% lipids, 48% sugars), Spirulina platensis (8% lipids, 60% sugars) and Chlorella sp. (19% lipids, 56% sugars) [ 35 , 36 ]. Today nearly 10 countries are keen in biofuel production from the algae biomass. Various techniques applied for the processing of microalgal biofuels is given in Fig. 4 .
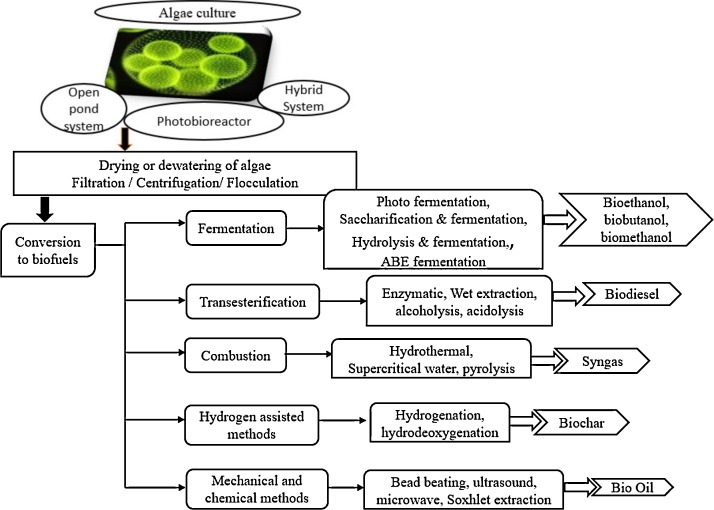
Steps and techniques involved in biofuel production using microalgae.
3. Algae – Growth, extraction and conversion
During the last decade considerable attention is drawn by algae for the economic possibilities in their mass growth. The biofuel synthesis from algal biomass proceeds through the following steps.
Culturing of algae
Harvesting of algae (or) Dewatering of algae
Extraction of oil from algae
Purification of algal oil
Processing of oil into biofuels
3.1. Culturing of algae or algaculture
Algaculture refers to the growth of algae similar to that of aquaculture. The growth expectation of algae is very simple and affordable i.e. sufficient light, naturally available dissolved nutrients and CO 2 [ 37 , 38 ]. Unialgal growth without contamination of other eukaryotic or prokaryotic organisms and axenic culture (bacteria free) is challenging. The growth rate of algae is spectacularly high (doubling by 24 h) unlike plants as their energy is not spent on the growth of their parts. The two kinds of algae culture classified based on the growth characteristics are:
Batch culture (BC): The inoculation of algal cells in a container when the abundant resource is available follows the sigmoidal curve. The loss of medium slays the culture and this can be subdued by introducing small volumes of fresh medium into existing culture.
Continuous flow culture (CFC): The regulated addition of adequate volume of fresh medium rich in nutrients (infinite source) to the culture medium to attain “steady state” is performed in CFC method. A steady state is the uniform cell density where birth rate is equivalent to death rate. This is done proportional to the growth of algae in a special culture technique known as “Turbidostat culture or Chemostat culture”. Turbidostat culture is the fresh addition of medium to the culture when the growth reaches a certain limit while Chemostat culture is the introduction of fresh medium to the culture at a predetermined rate.
Major physical parameters that affect the growth of algae are:
pH: The total collapse of algal cell wall occurs with the unoptimized pH level. The proper cell growth happens in the pH range of 8.2–8.7 and supplement of CO 2 into the medium enables the attainment of optimized pH.
- (a) Fluoroscent lamps: The radiation in 380–500 nm (blue light) and 600–700 nm (red light) is preferred for algal growth.
- (b) Photoperiod: The illumination period is expected to be around 16–18 h for the appropriate culture maintenance
- (c) Light intensity: Algae growth differs with the intensity of light ranging from 5% to 10%. Mostly, the light/ dark cycles are followed as the cells do not grow in continuous illumination.
Temperature: The temperature of the culture medium varies with respect to the temperature zones of regions. Algaculture in countries like India and US (temperate zones) operates at 10 °C–25 °C and in tropical countries (ex., Brazil and Singapore) the temperature of action is below 20 °C. The temperature beyond 35 °C leads to destructive algal growth.
Medium of culture: The medium is responsible for the contamination of culture and this creates hindrance in sterilization of culture. The quality of water used in media has significance and sea water with unpredictable contaminants is a serious issue in culturing medium. The sea water may contain vitamins, chelating agents, buffers, soil extract etc.,
Three major types of culturing are practiced worldwide and are discussed in this section.
3.1.1. Open pond system
Algae usually grow in lakes and copying this similar pattern known as open pond system (OPS) is used for algae culturing. The ponds are of one-foot depth and algal cultivation could be from one acre to several acres. OPS is the most common system used in the algal growth. The types of OPS are: Raceway ponds, natural ponds (shallow lagoons and shallow ponds), mixed ponds, circular open ponds mixed with center pivot mixer. The lack of agitation and low sunlight penetration in the open pond system results in inadequate mass and heat transfer. Whereas the raceway pond system (RPS) is a cost cutting mechanism made with concrete earth. In RPS, a closed loop with recirculation channel (03.m deep) is designed with paddles for better mixing, laminar flow and circulation of CO 2 . But it also has high peril of contamination with low rates of production due to its sensitivity to environmental fluctuation. Perhaps, they are easy to maintain and operate [ 39 ]. Narala et al. [ 40 ] studied the biofuel extraction from Tetraselmis sp. M8 by OPS and by 32nd day about 2.8 × 10 6 cells/mL was harvested.
3.1.2. Photobioreactors (PBR) -Closed loop culturing
Intensive research on algae production compelled the idea of closed reactor systems. Photobioreactor (PBR) is a worth substitute for OPS for its massive productivity rate and high quality of algae. Researchers have created many versions like tubular, bubble, Christmas, plate, horizontal, foil and porous PBRs. The TBR is the common PBR type used in the algaculture and it comprises of tubular solar arrays, biomass unit, exchange column to exchange gas and pump. The vertical column TPBR (VTPBR) offers good gas exchange while the horizontal column TPBR (HTPBR) gets better access of light and also possess high surface area. However, the negatives of the VTPBR is low surface to volume ratio and HTPBR are low mass transfer leading to difficulty in CO 2 elimination, and excessive heat generation. Disappointingly, in general PBRs suffer from their high capital cost which exceeds the output due to its complexity and exclusive erection materials [ 41 , 42 ]. Further, it suffers from a) improper CO 2 and O 2 balance b) Control in temperature and c) biofilm formation (Fouling).
3.1.3. Hybrid systems
Phototrophic system is considered to be economical as the alga grows using CO 2 and sunlight. On the other hand, a very slow rate of growth is witnessed. The energy source is replaced by a carbon rich compound or biosugars like glucose, dairy products and waste food items in hetrotrophic systems. This receives bright focus in biofuel industry as the algae grown in this environment yields better biodiesel. Adhering to the cost and energy constraints, the proposals of hetrotrophic mode is restricted. Screening among the two methods becomes a difficult task and hence a hybrid cultivation needs to be developed [ 43 ]. Synergizing the effectiveness of OPS and PBR can be achieved by the hybridization of both systems. The two stage hybrid cultivation system is the advanced version in the algaculture where the cell medium is transferred from OPS to RPS when the nutrients are found to decline. The feasible separation of biomass from the lipid accumulation and least possibility of contamination strikes the positive note. The hybrid system can be of a) small PBRs with big ponds and b) ponds with large PBRs. The hybrid system comprises of two stages:
PBRs are chosen as I phase to reduce the lipid accumulation and contamination in the culture. The density of biomass can be increased with the closed PBRs.
The selection of OPS in the II stage increases the economic compatibility of the
process. The two most significant at the II phase after the completion of I phase helps to promote rich carbohydrate and lipids in algae.
3.1.3.1. Increase of lipid content by alternative nitrogen supply
The trial of reducing the nitrogen environment retards the growth of culture. The latest research shows the supply of nitrogen at the beginning of the culture growth and deprivation of the same after the considerable dense biomass raises the lipid production [ 44 ]. The nitrogen starvation disrupts the cell and directs the carbon towards carbohydrate and lipid production. Nannochloropsis gaditana and Chlorella protothecoides are the few algae that produced good results in the switch over of nitrogen source.
3.1.3.2. Brine condition
This methodology is reverse to the nitrogen supply mechanism. Better products were produced only with two stage cultivation process compared to single stage cultivation. Enhancement of algal growth was observed with low saline condition and the gradual increase of salinity lowered the metabolism. The lower salinity level improved the lipid and carbohydrate generation in algae. Chlorophycae species indicated the effect of salinity in their growth in II stage cultivation [ 45 , 46 ].
3.2. Harvesting or de-watering or algae
The cultured algae needs to be dewatered in order to access the lipid profile. The dewatered algae looks like an interim of solid-liquid medium instead of a liquid which flows easily. The experiments prove that only 0.1% of dry matter is available in 1 L of cultured media. Filtration and centrifugation are the processes involved in removing water from algae. Many advanced mechanics are explored under these categories. Flocculation and membrane filtration is effective in drying algae [ [47] , [48] , [49] ]. Methods involved in filtration: Pressure, Vacuum, deepbed sand, cross flow and magnetic filtration.
3.3. Algal oil trough lipid extraction from dry algal mass
The biological micro species has multilayered cell wall made of polysaccharides and cellulose synthesized from silicic acid. The cell wall envelops the lipids or fatty acids and the removal of algal oil is known as lipid extraction. The specific extraction of lipids is also performed by solvent extraction using methanol and chloroform. Interest is on microwave, grinding, bead beating and ultrasound mechanical methods for extraction. This method does not require extra chemicals and the subsequent extraction step becomes easier. Mostly, bead beating is done to disturb the cell walls of microbes in small scale level with beads made up of ceramics or glass. The beads of large surface area are mixed with the cell suspension and their vigorous shaking collapses the walls to release the lipids. Pulverizing the dried algal biomass is analogous to wheat milling which protects the maximum nutrient content of the material. In recent years, research is directed towards extraction free from solvents. The super critical fluid technique (SCF) accomplishes the demand by producing safe and good quality end products. The efficacy of this method in extracting specific components from a complex biological species is worth enough.
The oil extracted using n-heptane by Soxhlet extraction method is much lower than SCF method. About 39.4% of oil was extracted from algae using Cyclopentyl Methyl Ether (CPME) and ethanol (EtOH) as Super critical fluids against 32.8% of usually used CO 2 [ 50 ]. The obtained algal oil is a pure triglyceride and has to be upgraded by reduction catalysts to liquid fuels. Algal oil was extracted from the algae collected from Simlapuri Nahar, Ludhiana, Punjab. 9 wt% and 8 wt% of oil was gained using costly hexane and inexpensive acetone respectively [ 51 ]. Lipids extracted from various microalgae and the method of application is given in Table 2 . Supercritical Water Reactor (SCWR) for extraction of algal oil. The research article concentrates on the demand for liquid transportation fuels and the further sections will elaborate on the routes to synthesize liquid fuels in specific.
Lipid extraction from algae [ [52] , [53] , [54] , [55] ].

4. Unprocessed algal oils as biofuels
The concept of using oil of vegetables without any type of conversion and processing as fuel in the engine emerged in Paris by 19th century when Rudolph Diesel made use of groundnut oil to operate the engine [ 56 ]. However, the application of raw oils as such in engines is technically unfeasible and poses lot of problems in the long run because a) it is highly viscous, possess low cetane value and low flash point, b) it causes plugging and gumming of filters, c) it causes engine knocking and d) it deposits carbon on piston. Hence a suitable technique is required to convert the vegetable oil into a sustainable biofuel with good fuel characteristics and only such fuel can be an alternative to fossil fuels. Engine performance was evaluated using unprocessed raw algal oil in a digital software Diesel RK which was analogous to Yanmar diesel engine by Tsaousis et al. [ 57 ]. The power generation of the fuel which magnifies the consumption of fuel, spikes the CO 2 emissions but indeed relates to lower emissions of NOx.
4.1. Techniques towards novel fuels
The journey of making biofuel is not a recent work perhaps was kicked on by mid of 18th century itself. Although the biofuel was introduced during II world war for the production of glycerol and its application as explosives, its first commercial patent was filed by late 19th century by a Brazilian researcher, Expedito Parente. This section will discuss the procedures followed by researchers to convert biomass into alternative fuels ( Fig. 5 ). The productive value of oil from biomass grown in lands interprets the superiority of algae over the other food crops. Algae yields liberal quantity of oil compared to the common oil supplying crops and a better aspect of alga in biofuel generation can be understood. ( Fig. 6 ).
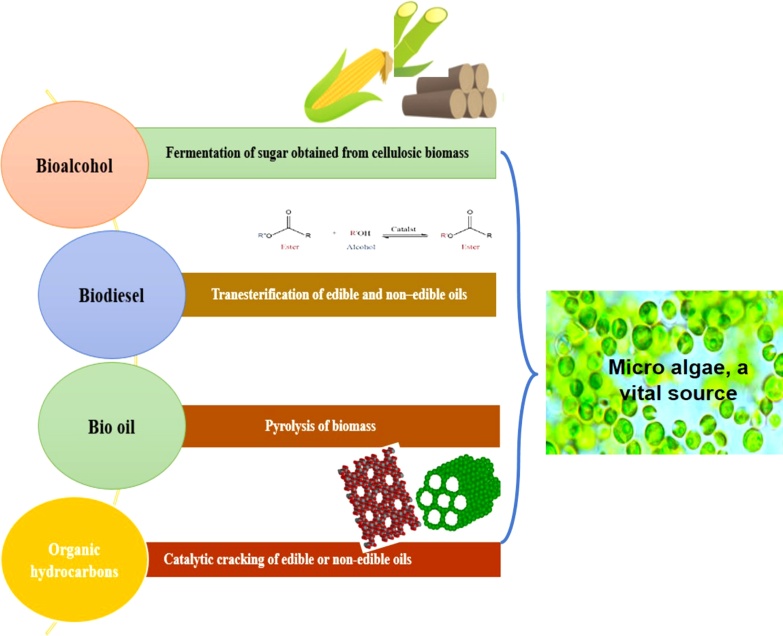
Techniques involved in the production of biofuel.
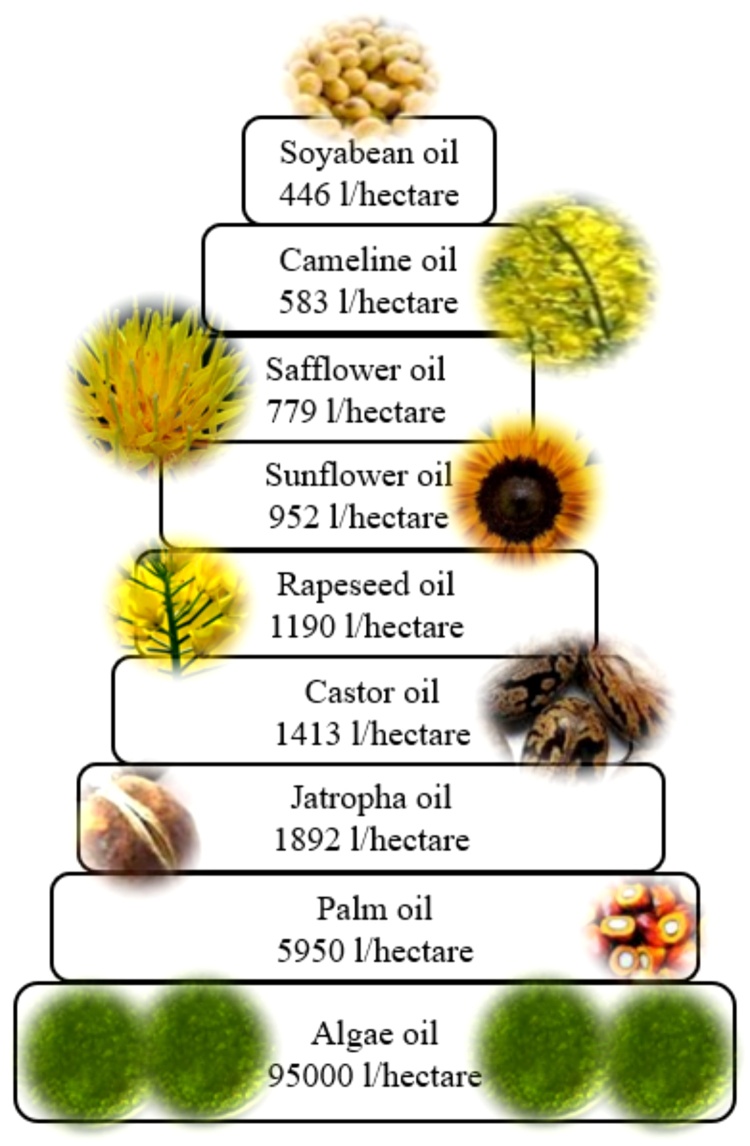
Oil yielding capacities of different biomass.
4.1.1. Fermentation
Ethanol derived from vegetables is considered as the cleanest liquid fuel [58, 59 ]. The conversion of biomass to ethanol is done through a technique called fermentation. Sugar cane and starch containing materials are used for the production of ethanol on commercial scale. This process involves a) solublization of starch (liquification step), b) conversion of soluble starch into glucose (hydrolysis step) and c) conversion of glucose into ethanol (fermentation step). The process involves crushing of biomass, addition of water and yeast and fermenting them in the large tanks called fermentors.
4.1.1.1. Fermentation in synthesis of algae biofuel
Each cell of the algae is a rich source of ethanol factory. The outer cell walls of algae constitute of pectin and alginate while the nucleus of the green algae is made of hemi cellulose or polyose with nearly 3000 sugar units and cellulose (polysaccharide) made by 15,000 sugar molecules. The different species of algae has potential carbohydrate content which on cell lysis turns to be feasible feedstock as bioethanol. Chlamydomonas reinhardtii and Chlorella vulgaris are spotted to have surplus carbohydrate and the estimated bio ethanol production from algae by US Renewable Fuels Standard is 36 billion gallons [ 60 , 61 ]. The complex form of carbohydrate in algae has to be broken to monomers prior to fermentation step and is followed by fermenting by using specific microorganisms like bacteria or yeast at approximately 38 °C [ 62 ]. Scenedesmus dimorphus is an apt alga to produce ethanaol with 53 W/W of carbohydrate. Scientist reported sugars stemmed from Scenedesmus sp. resulted in 93% yield of bioethanol. In the heterolactic fermentation, choice of fermenter microbes facilitates the fermentation of algae ex. Saccharomyces cerevisiae [ 63 ]. The energy derived from bio alcohol by fermentation was observed to be as low as 1 because half of the cost of production of bio alcohol is spent in the distillation of bio alcohol from the fermentation mixture. Fermentation faces the challenge during scale up and also commercialization of this fuel is solely dependent on the source being used for the purpose. The process is also claimed to be lengthy and is found to be time consuming extending from several hours to days. Although bioethanol is clean, produces less CO, hydrocarbons and oxides of N 2 , it is an oxygenated fuel containing 35% of oxygen which on combustion often produces aldehyde which is the culprit for photochemical smog. In addition, the production cost of enzyme makes it uneconomical.
4.1.2. Transesterification
The front runners in synthesizing biodiesel by tranesterification process under EPA Renewable Fuel Standard are Biodiesel International, Biosource Fuels and Crown Iron Works Company located in US. The lipids of microalgae ranging from 20 to 50% and the conversion of this into C18 range carbon fuels by the process called transesterification is a desirable task. Tranesterification is the reaction between one mole of triglyceride molecule which is a complex ester and 3–4 moles of alcohol to produce simple esters (Biodiesel). The transesterification technique is often catalyzed by several acid catalysts namely sulphonic acid and sulphuric acid and base catalysts such as NaOH, KOH, sodium methoxide, sodium ethoxide and K 2 CO 3 . The base catalyzed reactions are favoured industrially because the base catalyzed process is less corrosive than acid catalyzed process. The acid catalyst add H + to carbonyl group producing a stronger electrophile whereas the base catalyst eliminates a proton from the alcohol rendering a stronger nucleophilic. Methyl and ethyl esters (biodiesel) are obtained by using methanol and ethanol respectively. To overcome the problems posed by homogenous catalysts, transesterification is prompted through heterogeneous catalysis path. The silica alumina framework zeolites (MOR, HY, HZSM-5, Hβ, materials with large pore size like mesoporous materials (MCM-41, SBA-15, MCM-48), metal oxides (ZrO 2 , WO 3 , CaO, ZnO, SrCO 3 ) and hetropoly acids (H 3 PW 12 O 40 , H 4 SiW 12 O 40 ) have been in use over a decade [ 56 , 64 , 65 ]. Food crops, vegetable oils, animal fats and several other sources were the targets for conversion into biodiesel.
4.1.2.1. Biofuel synthesis from algae by transesterification process
Algae competes the fellow contestants in the biodiesel synthesis market which gives a insight for future oil demand. The conversion of various feedstocks to biodiesel is compared in Fig. 7 . It has to be noted that, catalysts plays a vital role in the biodiesel forming reaction irrespective of the type of oil examined. But, algal oil displays a remarkable tendency to get converted into diesel range esters. With several classes of catalysts in action, porous catalyst Hβ and mixed oxide of Nickel and Molybdenum turns to be veracious materials. The biodiesel yield in the presence on these catalysts almost reaches 100%. Liu et al. [ 66 ] extracted the lipids from microalgal strain by cautious engulfing of them by newly synthesized nanoparticles in mesoporous range. The transfer of lipids present within the cell was enabled by in vitro method with SrO 2 /CaO intrusion into the cells. Potential of nano carbon particles is also being used to convert lipids onto biodiesel. The conventional transesterification uses inorganic catalysts and has a demerit of polluting the environment due to its disposal hitches. Therefore, green substitutes like enzymes can act as better auxiliary. Biological catalysts are also treated for the biodiesel formation and out of them lipases have created a niche in the industry [ 67 , 68 ]. Immobilized lipases on metal oxide nanoparticles have fine thermal stability, corresponds to good selectivity and also can be easily separated. Biodiesel yield was high as 90% with enzyme concentration (1%–3.5%). Croto megalocarpus when catalyzed by sulphated tin oxide over silica yielded 95 % of biodiesel [ 69 ]. Lipase R oryzae immobilized on exchangeable resins transesterified pistachia chnesisBge into 94% biodiesel [ 70 ]. Many countries have begun to fuel their future with biofuels and their native plants were chosen as major feeds. In 2018, United States (380888 TMT) is in the spotlight followed by Brazil (21375 TMT) and Indonesia (4849 TMT) ( Fig. 8 ). To list a few companies working on the algal diesel are Terravia Holdings (Formerly called as Solazyme), Algenol, Blue Marble Production, Culture Biosystem Organization, Oil Inc., Proviron industries, Solix Biofuel, Reliance Life Science.Algenol is a massive firm located in Florida with a bouncing stock value of $3.1 million produces 8000 gallons of biodiesel for every acre of algal harvest annually. Reliance from India operates to deliver 100 barrels of biodiesel per day. Works in biofuels are established in developing countries like India and a joint venture is commenced by Williamson Magor Bio Fuel Limited (North East India) and Oils of U.K. The composition and characteristics of biodiesel from jatropha oil through transesterification process with the correlated values of different standards [ 71 ] ( Table 3 ).
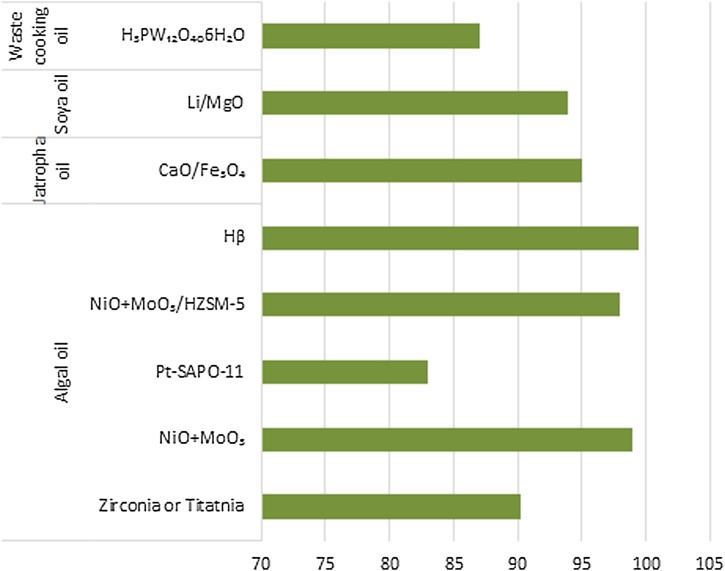
Transesterification of feedstocks to biodiesel.
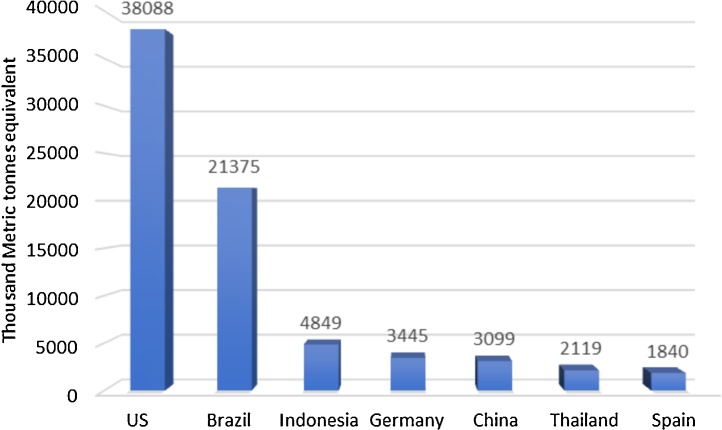
Leading countries in Biodiesel production.
Properties of Jatropha FAME (fatty acid methyl ester or Biodiesel).
The process produces large quantity of fuel within a short time and the quality of the product is also impeccable. Despite of few merits, to obtain a maximum yield of biodiesel, the alcohol has to be used in large quantity. Literature study reveals that the oil to alcohol ratio varied from 1:3 to 1:9 [ 72 ] The excess of alcohol favours the formation of the mixture of fatty acid alkyl esters (biodiesel) but the excessive amount of alcohol makes the revival of glycerol tricky. Among the base catalysts, sodium methoxide was found to be the most active catalyst (98% yield, reaction time – 30 min) even if its molar ratio is as low as 0.5 mol%. The base catalyst requires absence of water and hence it is inappropriate for typical industrial processes. The transesterification process is also catalyzed by Bronsted acids such as RSO 3 H and H 2 SO 4 . This process produces high yield of alkyl esters but the reaction is very slow. Both the acid and base catalyzed transesterification process requires longer time, consumes larger quantity of methanol or ethanol and hence they are not cost effective. The usage of biodiesel in the engines is restricted and hence has to be blended with the diesel or petrol [ 73 ] and it also burns emitting smoke as gums are formed on engines [ 74 ].
4.1.2.2. Engine performance using algal biodiesel
The importance of running towards these alternative fuels lies in its application in the available design of engine. The algal biodiesel’s application in single cylinder engine at 1500 rpm provides the lucidity it against diesel fuel. The properties like fuel usage, thermal capacity, emission profile were much similar [ 75 ]. Algal oil blended with diesel fuel in 20% ratio completely reduced hydrocarbon exhaust and was claimed to be perfect alternative for diesel engine [ 76 ]. Naresh and Prabhakar [ 77 ], also reported the exact 20% blending of algal oil ended with better emission characteristics strikingly it has to be noted most of the research papers defends blending of diesel with 20% algal oil [ 78 ]. Biodiesel yield from N. cinta with B10 and B7 blending pattern was of good cetane value (50–51) [ 79 ].
4.1.3. HTL of algal biomass
Hydro thermal liquification (HTL) of wet biomass is a promising way to thick and viscous bio crude rich in energy density compared to combustion process. HTL converts entire algae nutrients including proteins and carbohydrates and not only lipids. Therefore, the lipids augmentation step for this technique is bypassed. The operational cost of this technique is expected to be more and this reduces the upgradation of this method. Besides, the possibility of processing the algae with high water content (90%) without any pre-treatment should definitely make the expenditure for this method low than the drying cost of algae. HTL in the absence of a catalyst has a low conversion rate while the addition of catalyst creates a hype in conversion rate. A whole wet microalgae can be liquified in a pressurized condition of 25 MPa and at a temperature below 375 °C. Vinod kumar et al. compared HTL of macroalgal blooms with and without catalyst. Catalytic HTL (Na 2 CO 3, TiO 2 ) showed double conversion (20%) compared to noncatalytic HTL. HTL of Nannochloropsis (NAS) at a 300 °C with Ni doped TiO 2 yielded 48 % of biocrude at 90 % conversion order. The complete combustion of algae released higher % of NO x into atmosphere due to the significant presence of nitrogen in algae (5−8 wt%) [ 80 ].
Biocrude from HTL is found to be low in HHV (32−35 MJ/Kg) and high in oxygen content (10–11 wt%). The viscosity of the fuel obtained by this technique has low viscosity and also had high concentration of lower hydrocarbons [ 81 ]. The engine run with this biocrude will result in NO x and SO X emissions from the exhaust [ 82 ]. Apart from that, the engine performance also will be low owing to their low calorific value. However, HTL biocrude used in the diesel run marine engines with low speed will have trace emissions.
4.1.4. Pyrolysis
Pyrolysis involves the direct thermal decomposition of the biomass at high temperature (400 °C – 1000 °C) in the absence of catalyst and oxygen to produce bio-oil into solid (char and coke), liquid (bio oil) or gaseous fuels (methane and higher gaseous hydrocarbons) in a limited supply of oxygen [ 83 ]. Two types of pyrolysis are reported in the literature: slow (low process temperatures and longer time) and fast pyrolysis (higher process temperatures and shorter time) [ 84 ]. The product produced through the pyrolysis technique is called bio oil. An aqueous phase comprising of varied low m.w compounds (primary alcohol, acid and ketone) and non-aqueous phase of oxygen containing compounds (aliphatics, alcohols, carbonyls, acids, phenols and cresols etc.) and aromatic hydrocarbons [ 85 , 86 ]. Several research works were reported on the thermal treatment of different vegetable oils like sunflower, safflower, palm, castor, tung oils [ [87] , [88] , [89] ]. The comparison between the characteristics of pure soyabean and pyrolyzed soyabean oil is given
in Table 4 [ 90 ].
Comparison of fuel properties of pure soyabean oil with pyrolyzed soyabean oil
The table reveals that there is a drastic reduction in viscosity and pour point of the bio oil derived in the pyrolysis of soyabean oil. However, no significant difference in the heating value between raw and pyrolysis oils were noticed. The different types of biomass cracked thermally in fluidized bed reactor is given in Table 5 . The products derived the biomass are distinct from other sources.
Thermochemical conversion of crop oil and lignocellulosic biomass.
4.1.4.1. Pyrolysis of algal biomass to produce biofuel
The combustion of biomass emits CO 2 which in turn 183 tonnes of CO 2 is absorbed by 100 tonns of algae for its growth and thus self-sustaining the CO 2 cycle. Thus, algae helps in greenhouse gas sequestration [ 96 , 97 ]. Innumerous articles published on pyrolysis of algae is a supplement for aspirants on algal fuel [ [98] , [99] , [100] , [101] ]. Yanik and Sinag pyrolyzed algae Laminaria digitate and fucus serratus at 500 °C in fluid reactor [ 102 ]. Products from the process were solids, liquid and gaseous in nature. Bio oil extracted from the algae was 17%. Pyrolysis of algae yield lower hydrocarbons range of liquids, syngas, bio oil and bio char. 44% of bio oil was reported in the pyrolysis of algal biomass from waste water treatment plant [ 103 ]. Nannochloropis gaditana , a macro algal biomass produced bio oil with highest calorific value of 12.6 MJ/Kg. Majority of the liquid product were decanes and gas products restricted to methane [ 104 ]. About 78% of bio oil was a promising result in flash pyrolysis of macroalgae [ 105 ]. Open pond lake system grown algae on cracking at 500 °C delivered 59% of bio oil with 21 MJ/Kg of heating value [ 106 ]. The slow pyrolysis process always lead to low bio oil contrast to the fast pyrolysis [ 107 ]. The pyrolysis done at higher temperature lacks the efficiency to achieve the products specifically.
The various strains of algae produced different results and the temperature at which pyrolysis was performed also played a vital role ( Table 6 ). Catalytic hydrocracking is another technique in which the compounds with high molecular weight (biomass) are broken down to form low molecular weight compounds using suitable catalysts and stream of hydrogen (1−10 MPa) in the temperature range of 200 °C–450 °C [ 125 ]. Cracking and hydrogenation are complementary, where the cracking of biomass yields olefins by absorbing heat energy (endothermic) and hydrogenation provides heat for cracking (exothermic). The extracted algal oil can be converted into useful fuels within a prescribed range of hydrocarbons and this conversion was mediated by both homogeneous and heterogeneous catalytic way. The homogeneous catalysts used in transesterification cracked the oil but the catalysts impacts the acidity of the medium enhancing the chance of engine corrosion. Catalytic cracking of algal oil and vegetable oils are intensively studied in the production of modest fuels. HZSM-5, a zig zag channeled microporous catalyst produced bio oil yield of 52.7 wt% with microalgae. The aromatic content in the biofuel was nearly 26 wt% [ 126 ]. Upgrading of oil catalytic cracking of 100 Kg of algae performed in ASPEN plus was 95% successful with 41% kerosene yield. Coprocessing of biofuel derived from HTL (hydro thermal liquification) of algae combined with HVGO (Heavy vehicle gas oil) decreased the conversion rate and also increased coking of catalyst. This also further confines the economic suitability of algal oil blended with HVGO [ 127 ]. Fuels in used for aviation or avgas, a highly refined version of gasoline was obtained cracking of alga at H 2 atmosphere in presence of Pt-Re catalyst. About 50% of Bot-oil (Botryococcus braunni ) cracking yielded 16.7% of C10 -C15 range products which could be termed as diesel [ 128 ]. Zhao et al. [ 129 ] catalytically deoxygenized microalgae with non-sulfide metal as catalysts into hydrocarbons.
Pyrolysis of Micro algae into bio oil.
The main advantages of this method are (a) it is inexpensive [ 130 ] (b) the bio oil is easy to store, transport and (c) fuels of high demand can be easily prepared by upgrading the oil [ 131 ]. While the drawbacks of bio oil are (a) highly viscid, harsh and lacks thermal stability [ 132 ], (b) it exhibits low calorific value and (c) resemblance to the reactant oil as it has predominant oxygenated molecules [ 133 ].
4.2. Algae- the new hope of future energy but a long way to go
The microorganism have the potential to yield higher quantity of biofuel compared to other general biomass. Interestingly, algae is a treasure hunt for the society to develop butanol, ethanol and jet fuels. The algal bloom is definitely easy to grow in an open pond or closed loo systems in unfertile lands. The algal oil is inedible and therefore do not have to answer for food availability controversies. The higher concentration of lipids/ fatty acids present in algae enables its successful conversion into biofuel. The hypes for algal biofuel is quite acceptable and but also has to accept few of the discontents. Companies like Algenol, Solazyme and Sapphire Energy attracted 100’s of millions of dollars from private sectors. With a promise to produce millions if gallons of fuel within short span. The large span invested were in vain as the industrial goals were not attained. In 2017, Professor Kevin Flynn, Swansea University reported that to equalize 10% of fuels utilized in European transportation, the algae should be cultivated in ponds thrice the size of Belgium. Furthermore, the fertilizers should be required in anomalous level approximately 50% of European crop plant needs. As a result, the companies shifted their target to prepare cosmetics and animal fodder regarding the huge investments and less fruitfulness of the project. Irrespective of failures in the research, commendable achievements has to be remembered.
5. Conclusion
Conventional fossil fuel usage makes the society to contemplate on producing the renewable and sustainable sources of energy. Exploitation of biomass is the right choice to produce biofuels with ultimate requisites of alternative fuels. Sources used in I and II generation biofuel are unviable and are criticized for the economic, social and food insecurity. Many countries have pinned their hope in bio III generation biofuel constituted of whole alga and oil derived from alga after conversion processes. Though the thought of alga cultivation is simple, however, the existence of difficulties in feedstock production with high lipid content and harvesting needs to be addressed. Challenges is really enthralling in the area of conversion of alga into perfect fuel. The algal biofuel works wonders in engines and yet a detailed study on the parameters for fuel compatibility is required. However, also, we have a long way to go for making algal biofuel a commercially viable alternate in place of fossil fuel.
Declaration of Competing Interest
The authors declare that they have no known competing financial interests or personal relationships that could have appeared to influence the work reported in this paper.
Contributor Information
Pham Anh Duc, Email: [email protected].
Arivalagan Pugazhendhi, Email: [email protected].
- 1. Peng L., Kiyoshi S., Hisao M. Extraction techniques in sustainable biofuel production: a concise review. Fuel Process. Technol. 2019;193:295–303. [ Google Scholar ]
- 2. Piotr B., Aneta B.-B., Elżbieta Jadwiga S., Krzysztof ózef J.J., Bogdan D., James W.D. Development of renewable energy sources market and biofuels in the European Union. J. Clean. Prod. 2019;228:467–484. [ Google Scholar ]
- 3. Curtin J., McInerney C., Gallachóir Ó B., Hickey C., Deeney P. Quantifying stranding risk for fossil fuel assets and implications for renewable energy investment: a review of the literature. Renewable Sustainable Energy Rev. 2019;116(109402) [ Google Scholar ]
- 4. Ting W., Boqiang L. Fuel consumption in road transport: a comparative study of China and OECD countries. J. Clean. Prod. 2019;(206):156–170. [ Google Scholar ]
- 5. EIA projects nearly 50% increase in world energy usage by 2050, led by growth in Asia. URL: https://www.eia.gov/todayinenergy/detail.php?id=41433 .
- 6. Lippman T.W. ABC-CLIO; 2019. Lippman Crude Oil, Crude Money: Aristotle Onassis, Saudi Arabia, and the CIA. [ Google Scholar ]
- 7. Maamoun N. The Kyoto protocol: empirical evidence of a hidden success. J. Environ. Econ. Manage. 2019;95:227–256. [ Google Scholar ]
- 8. Pascoal C.V.P., Oliveira A.L.L., Figueiredo D.D., Assunção J.C.C. Optimization and kinetic study of ultrasonic-mediated in situ transesterification for biodiesel production from the almonds of Syagrus cearensis. Renew. Energy. 2020;147(1):1815–1824. [ Google Scholar ]
- 9. Zdujić M.V., Veljović D.N., Krstić J.B., Banković-Ilić I.B., Veljković V.B., Stamenković O.S. Valorization of walnut shell ash as a catalyst for biodiesel production. Renew. Energy. 2020;147(1):1033–1043. [ Google Scholar ]
- 10. Manigandan S., Atabani A.E., Ponnusamy V.K., Pugazhendhi A., Gunasekar P., Prakash S. Effect of hydrogen and multiwall carbon nanotubes blends on combustion performance and emission of diesel engine using Taguchi approach. Fuel. 2020;279:118120. [ Google Scholar ]
- 11. Goga G., Singh Chauhan B., Mahla S.K., MukCho H. Performance and emission characteristics of diesel engine fueled with rice bran biodiesel and n-butanol. Energy Rep. 2019;5:78–83. [ Google Scholar ]
- 12. Sarno M., Ponticorvo E. A new nanohybrid for electrocatalytic biodiesel production from waste Amalfi coast lemon seed oil. Fuel. 2020;267(1):117178. [ Google Scholar ]
- 13. Thiyagarajan S., Herfatmanesh M.R., EdwinGeo V., Peng Z. Experimental investigation into the effect of magnetic fuel reforming on diesel combustion and emissions running on wheat germ and pine oil. Fuel Process. Technol. 2019;186:116–124. [ Google Scholar ]
- 14. Aditya K., Appa Rao V.B. Effect of fatty acid composition on the performance and emission characteristics of an IDI supercharged engine using neat palm biodiesel andcoconut biodiesel as an additive. Biofuels. 2019;10:591–605. [ Google Scholar ]
- 15. AnanthaRaman L., Deepanraj B., Rajakumar S., Sivasubramanian V. Experimental investigation on performance, combustion and emission analysis of a direct injection diesel engine fuelled with rapeseed oil biodiesel. Fuel. 2019;246:69–74. [ Google Scholar ]
- 16. Hazra M.A., Rasul M.G., Khan M.M.K., Ashwath N. Emission characteristics of polymer additive mixed diesel-sunflower biodiesel fuel. Energy Procedia. 2019;156:59–64. [ Google Scholar ]
- 17. Patel C., Tiwari N., Agarwal A. Experimental investigations of Soyabean and Rapeseed SVO and biodiesels on engine noise, vibrations, and engine characteristics. Fuel. 2019;238:86–97. [ Google Scholar ]
- 18. Sharma A., Kodgire P., Kachhwaha S.S. Biodiesel production from waste cotton-seed cooking oil using microwave-assisted transesterification: optimization and kinetic modeling. Renewable Sustainable Energy Rev. 2019;116(109394) [ Google Scholar ]
- 19. Lira T.A.M., Santos A.P., Moreti T.C.F., Lopes A., de Oliveira M.C.J., Neves M.C.T., Lamaguti P.S., De Lima L.P., Koike G.H.A., Silva R de A. Performance of agricultural tractor consuming diesel and biodiesel derived from babassu (’Orbinya martiana’) Aust. J. Crop Sci. 2019;13(7):1037–1044. [ Google Scholar ]
- 20. Espitia Cubillos A.A., Delgado Tobón A.E., Aperador Chaparro W.A. Oleoresin from copaiba as a raw material for the production of biodiesel. Int. J. Environ. Pollut. 2018;34(2):317–329. 2018. [ Google Scholar ]
- 21. Khandaln S.V., Banapurmathv R., Gaitonde N. Effect of exhaust gas recirculation, fuel injection pressure and injection timing on the performance of common rail direct injection engine powered with honge biodiesel (BHO) Energy. 2017;139(15):828–841. [ Google Scholar ]
- 22. Acharya N., Nanda P., Panda S., Acharya S. A comparative study of stability characteristics of mahua and jatropha biodiesel and their blends. Journal of King Saud University Engineering Sciences. 2019;31(2):184–190. [ Google Scholar ]
- 23. Sandouq A., Al-Hamamre Z. Energy analysis of biodiesel production from jojoba seed oil. Renew. Energy. 2019;130:831–842. [ Google Scholar ]
- 24. Ahmed T., Abdul M., Sahito R. Optimization of castor and neem biodiesel blends and development of empirical models to predicts its characteristics. Fuel. 2020;262(116341) [ Google Scholar ]
- 25. Samart C., Karnjanakom S., Chaiya C., Reubroycharoen P., Sawangkeaw R., Charoenpanich M. Statistical optimization of biodiesel production from para rubber seed oil by SO3H-MCM-41 catalyst. Arab. J. Chem. 2019;12(8):2028–2036. [ Google Scholar ]
- 26. Ogunkunle O., Ahmed N.A. Performance evaluation of a diesel engine using blends of optimized yields of sand apple (Parinari polyandra) oil biodiesel. Renew. Energy. 2019;134:1320–1331. [ Google Scholar ]
- 27. Kumar M.V., Babu A.V., Kumar P.R. Influence of metal-based cerium oxide nanoparticle additive on performance, combustion, and emissions with biodiesel in diesel engine. Environ. Sci. Pollut. Res. 2019;26:7651–7664. doi: 10.1007/s11356-018-04075-0. [ DOI ] [ PubMed ] [ Google Scholar ]
- 28. Arunkumar M., Kannan M., Murali G. Experimental studies on engine performance and emission characteristics using castor biodiesel as fuel in CI engine. Renew. Energy. 2019;131:737–744. [ Google Scholar ]
- 29. EU votes to scale back on biofuels linked to deforestation. Mongabay environmental news service and education platform. URL: https://news.mongabay.com/2015/04/eu-votes-to-scale-back-on-biofuels-linked-to-deforestation .
- 30. Second generation of biofuels could give forests a break. URL: https://www.climatechangenews.com/2012/10/03/second-generation-of-biofuelscould-give-forests-a-break .
- 31. Parente E. National Institute of Standards and Technology; 2007. Lipofuels: Biodiesel and Biokerosene. [ Google Scholar ]
- 32. Sani Y.M., Daud W.M.A.W., Abdul Aziz A.R. DOI: 10.5772/52790; 2012. Biodiesel Feedstock and Production Technologies: Successes, Challenges and Prospects. [ Google Scholar ]
- 33. Yi-Feng C., Wu Q. Chapter 17 - production of biodiesel from algal biomass: current perspectives and future. In: Pandey A., Larroche C., Ricke S.C., Dussap C.-G., editors. Biofuels. 2011. pp. 399–413. [ DOI ] [ Google Scholar ]
- 34. Mathimani T., Pugazhendhi A. Utilization of algae for biofuel, bio-products and bio-remediation. Biocatal. Agric. Biotechnol. 2019;17:326–330. [ Google Scholar ]
- 35. Demirbas A., Fatih Demirbas M. Importance of algae oil as a source of biodiesel. Energy Convers. Manage. 2011;52(1):163–170. [ Google Scholar ]
- 36. Xu L., Cheng X., Wang Q. Enhanced lipid production in Chlamydomonas reinhardtii by Co-culturing with Azotobacter chroococcum. Front. Plant Sci. 2018;9(741):754. doi: 10.3389/fpls.2018.00741. [ DOI ] [ PMC free article ] [ PubMed ] [ Google Scholar ]
- 37. Sasaki M., Takagi A., Ota S., Kawano S., Sasaki D., Asayama M. Coproduction of lipids and extracellular polysaccharides from the novel green alga Parachlorella sp. BX1.5 depending on cultivation conditions. Biotechnol. Rep. 2020;25:e00392. doi: 10.1016/j.btre.2019.e00392. [ DOI ] [ PMC free article ] [ PubMed ] [ Google Scholar ]
- 38. Rafiee P., Ebrahimi S., Hosseini M., WahTong Y. Characterization of Soluble Algal Products (SAPs) after electrocoagulation of a mixed algal culture. Biotechnol. Rep. 2020;25:e00433. doi: 10.1016/j.btre.2020.e00433. [ DOI ] [ PMC free article ] [ PubMed ] [ Google Scholar ]
- 39. Muraza O. Biodiesel production from algae by using heterogeneous catalysts: a critical review. Energy. 2014;78:72–83. [ Google Scholar ]
- 40. Narala R.R., Garg S., Sharma K.K., Thomas-Hall S.R., Deme M., Li Y., Peer M.S. Comparison of microalgae cultivation in Photobioreactor, open raceway pond, and a two-stage hybrid system. Front. Energy Res. 2016;4(29) [ Google Scholar ]
- 41. Stephens E., Ross I.L., Mussgnug J.H., Wagner L.D., Borowitzka M.A., Posten C. Future prospects of microalgal biofuel production systems. Trends Plant Sci. 2010;15:554–564. doi: 10.1016/j.tplants.2010.06.003. [ DOI ] [ PubMed ] [ Google Scholar ]
- 42. Oncel S.S. Microalgae for a macroenergy world. Renew Sustain Energy Rev. 2013;26:241–494. [ Google Scholar ]
- 43. Nagappan S., Devendran S., Tsai P.C., Dahms H.U., Ponnusamy V.K. Potential of two-stage cultivation in microalgae biofuel production. Fuel. 2019;252:339–349. [ Google Scholar ]
- 44. Sun X., Cao Y., Xu H., Liu Y., Sun J., Qiao D. Eff ;ect of nitrogen-starvation, light intensity and iron on triacylglyceride/carbohydrate production and fatty acid profile of Neochloris oleoabundans HK-129 by a two-stage process. Bioresour. Technol. 2014;155:204–212. doi: 10.1016/j.biortech.2013.12.109. [ DOI ] [ PubMed ] [ Google Scholar ]
- 45. Benavente-Valdés R., Aguilar C., Contreras-Esquivel J., Zavala A., Montañez J. Strategies to enhance the production of photosynthetic pigments and lipids in chlorophycae species. Biotechnol. Rep. Amst. (Amst) 2016;10:117–125. doi: 10.1016/j.btre.2016.04.001. [ DOI ] [ PMC free article ] [ PubMed ] [ Google Scholar ]
- 46. Sharma A., Arya S.K. Hydrogen from algal biomass: a review of production process. Biotechnol. Rep. 2017;15:63–69. doi: 10.1016/j.btre.2017.06.001. [ DOI ] [ PMC free article ] [ PubMed ] [ Google Scholar ]
- 47. Kwan T.A., Zimmerman J.B. Mono- and poly-unsaturated triacylglycerol fractionation from Chlorella sp. using supercritical carbon dioxide. Algal Res. 2019;43(101644) [ Google Scholar ]
- 48. Chen C.L., Chang J.S., Lee D.J. Dewatering and drying methods for microalgae Dry. Technology. 2015;33(4):443–454. [ Google Scholar ]
- 49. Pandey A., Pathak V.V., Kothari R., Black P.N., V V Tyagi V.V. Experimental studies on zeta potential of flocculants for harvesting of algae. J. Environ. Manage. 2019;231:562–569. doi: 10.1016/j.jenvman.2018.09.096. [ DOI ] [ PubMed ] [ Google Scholar ]
- 50. Santoro I., Nardi M., Benincasa C., Costanzo P., Giordano G., Procopio A., Sindona G. Sustainable and selective extraction of lipids and bioactive compounds from microalgae. Molecules. 2019;24(23):4347. doi: 10.3390/molecules24234347. [ DOI ] [ PMC free article ] [ PubMed ] [ Google Scholar ]
- 51. Karmakar R., Rajor A., Kundu K., Kumar N. Production of biodiesel from unused algal biomass in Punjab. India. Petroleum Science. 2018;15:164–175. [ Google Scholar ]
- 52. Ellison C.R., Overa S., Boldor D. Central composite design parameterization of microalgae/cyanobacteria co-culture pretreatment for enhanced lipid extraction using an external clamp-on ultrasonic. Ultrason. Sonochem. 2019;51:496–503. doi: 10.1016/j.ultsonch.2018.05.006. [ DOI ] [ PubMed ] [ Google Scholar ]
- 53. Onumaegbu C., Alaswad A., Rodriguez C., Olabi A. Modelling and optimization of wet microalgae Scenedesmus quadricauda lipid extraction using microwave pre-treatment method and response surface methodology. Renew. Energy. 2019;132:1323–1331. [ Google Scholar ]
- 54. Yusuff A.S. Energ Sources Part A; 2019. Extraction, Optimization, and Characterization of Oil From Green Microalgae Chlorophyta species. [ Google Scholar ]
- 55. Sovova H., Stateva R.P. New developments in the modelling of carotenoids extraction from microalgae with supercritical CO2. J. Supercrit. Fluids. 2019;148:93–103. [ Google Scholar ]
- 56. Sani Y.M., Wmaw D., Aziz A. Solid acid-catalyzed biodiesel production from microalgal oil the dual advantage. J. Environ. Chem. Eng. 2013;1(3) [ Google Scholar ]
- 57. Tsaousis P., Wang Y., Roskilly A.P., Caldwell G.S. Algae to energy: engine performance using raw algal oil. Energy Procedia. 2014;61:656–659. [ Google Scholar ]
- 59. Ho S.H., Huang S.W., Chen C.Y., Hasunuma T., Kondo A., Chang J.S. Bioethanol production usingcarbohydrate-richmicroalgaebiomassasfeedstock. Bioresour. Technol. 2013;135:191–198. doi: 10.1016/j.biortech.2012.10.015. [ DOI ] [ PubMed ] [ Google Scholar ]
- 60. Qari H., Rehan M., Nizami A.S. Key issues in microalgae biofuels: a short review. Energy Procedia. 2017;142:898–903. [ Google Scholar ]
- 61. Fivga A., Speranza L.G., Branco C.M., Ouadi M., Hornung A. A review on the current state of the art for the production of advanced liquid biofuels. Aims Energy. 2019;7(1):46–76. [ Google Scholar ]
- 62. Khan M.I., Lee M.G., Shin J.H., Kim J.D. Pretreatment optimization of the biomass of Microcystis aeruginosa for efficient bioethanol production. AMB Express. 2017;7:19. doi: 10.1186/s13568-016-0320-y. [ DOI ] [ PMC free article ] [ PubMed ] [ Google Scholar ]
- 63. McNeff C.V., McNeff L.C., Yan B., Nowlan D.T., Rasmussen M., Gyberg A.E. A continuous catalytic system for biodiesel production. Appl. Catal. A General. 2008;343:39–48. [ Google Scholar ]
- 64. Borges M., Díaz L. Recent developments on heterogeneous catalysts for biodiesel production by oil esterification and transesterification reactions: a review. Renewable Sustainable Energy Rev. 2012;16(5):2839–2849. [ Google Scholar ]
- 65. Liu X., Piao X., Wang Y., Zhu S., He H. Calcium methoxide as a solid base catalyst for the transesterification of soybean oil to biodiesel with methanol. Fuel. 2008;87:1076–1082. [ Google Scholar ]
- 66. Yuzbasheva G., Mostova P., Lomonosova B., Sineokii V. Biodiesel production via enzymatic catalysis. Appl. Biochem. Microbiol. 2014;50:737–749. [ Google Scholar ]
- 67. Thangaraj B., Solomon P.R., Muniyandi B., Ranganathan S., Lin L. Catalysis in biodiesel production—a review. Clean Energy. 2019;3(1):2–23. [ Google Scholar ]
- 68. Kafuku M.K., Kafuku G., Kansedo J., Lee K.T., Mbarawa M. Croton megalocarpus oil: a feasible non-edible oil source for biodiesel production. Bioresource Technol. 2010;101(18):7000–7004. doi: 10.1016/j.biortech.2010.03.144. [ DOI ] [ PubMed ] [ Google Scholar ]
- 69. Yu V., Xu Y., Xiao R. Lipases from the genus Rhizopus: characteristics, expression, protein engineering and application. Prog. Lipid Res. 2016;64:57–68. doi: 10.1016/j.plipres.2016.08.001. [ DOI ] [ PubMed ] [ Google Scholar ]
- 70. Knothe G., Sharp C.A., Ryan T.W. Exhaust emissions of biodiesel, petrodiesel, neat methyl esters and alkaline in a new technology engine. Energy Fuels. 2006;20:403–408. [ Google Scholar ]
- 71. Stumborg M., Wong A., Ed H. Hydroprocessed vegetable oils for diesel fuel improvement. Bioresour. Technol. 1996;56(1):13–18. [ Google Scholar ]
- 72. Chew T.L., Bhatia S. Catalytic processes towards the production of biofuels in a palm oil and oil palm biomass-based biorefinery. Bioresour. Technol. 2008;99(17):7911–7922. doi: 10.1016/j.biortech.2008.03.009. [ DOI ] [ PubMed ] [ Google Scholar ]
- 73. Raheman H., Ghadge S.V. Performance of diesel engine with biodiesel at varying compression ratio and ignition timing. Fuel. 2008;87(12):2659–2666. [ Google Scholar ]
- 74. Satputaley S.S., Zodpe D.B., Deshpande N.V. Performance, Combustion and Exhaust Emissions Analysisofa Diesel Engine Fuelled with Algae. Oil and Algae Biodiesel. 2018;5(1):23022–23032. [ Google Scholar ]
- 75. Velappan R., Sivaprakasam S. Study the performance of algae oil in diesel engine with various injection pressure. M. Int. Res. J. Eng. Technol. 2015;2(5):1152–1157. [ Google Scholar ]
- 76. Naresh V., Prabhakar S. Performance and emission characteristics of algae oil on VCR diesel engine. J. Chem. Pharm. Res. 2018;10(4):44–55. [ Google Scholar ]
- 77. Subramani L., Venu H. Evaluation of methyl ester derived from novel Chlorella emersonii as an alternative feedstock for DI diesel engine & its combustion, performance and tailpipe emissions. Heat Mass Transf. 2019;55:1513–1534. [ Google Scholar ]
- 78. Popovich C.A., Pistonesi M., Hegel P., Constenla D., Barnech Bielsa G., Martín L.A., Daminani M.C., Leonardi P.I. Unconventional alternative biofuels: quality assessment of biodiesel and its blends from marine diatom Navicula cincta. Algal Res. 2019;39(101438) [ Google Scholar ]
- 79. Kumar V., Kumar S., Chauhan P.K., Verma M., Bahuguna V. Low-temperature catalyst based Hydrothermal liquefaction of harmful Macroalgal blooms, and aqueous phase nutrient recycling by microalgae. Sci. Rep. 2019;9(11384) doi: 10.1038/s41598-019-47664-w. [ DOI ] [ PMC free article ] [ PubMed ] [ Google Scholar ]
- 80. Wang W., Xu Y., Xiaoxiao X., Zhang B., Tian W., Zhang J. Bioresource TechnologyHydrothermal liquefaction of microalgae over transition metal supported TiO2 catalyst. Bioresour. Technol. 2018;250:474–480. doi: 10.1016/j.biortech.2017.11.051. [ DOI ] [ PMC free article ] [ PubMed ] [ Google Scholar ]
- 81. Obeid F., Van T.C., Brown R., Rainey T. Nitrogen and sulphur in algal biocrude: A review of the HTL process, upgrading, engine performance and emissions. Energy Convers. Manage. 2019;181:105–119. [ Google Scholar ]
- 82. Demirbas A. Biomass resource facilities and biomass conversion processing for fuels and chemicals. Energy Convers. Manage. 2001;42:1357–1378. [ Google Scholar ]
- 83. Demirbas A. Biodiesel fuels from vegetable oils via catalytic and non-catalytic supercritical alcohol tranesterification and other methods: a survey. Energy Convers. Manage. 2003;44:2093–2109. [ Google Scholar ]
- 84. Bridgwater A.V. Catalysis in thermal biomass conversion. Appl. Catal. A Gen. 1994;116:5–47. [ Google Scholar ]
- 85. Meier D., Faix O. State of the art of applied fast pyrolysis of Lignocellulosic materials a review. Bioresour. Technol. 1998;68(1):71–77. [ Google Scholar ]
- 86. Araujo A.M.M., Lima A. de O., Gondim A.D., Juranci D., Luiz L.D., Souza A.Z. De. Thermal and catalytic pyrolysis of sunflower oil using AlMCM-41. Renew. Energy. 2017;101:900–906. [ Google Scholar ]
- 87. Angın D., Tiryaki A.E. Application of response surface methodology and artificial neural network on pyrolysis of safflower seed press cake. Energy Sources. 2016;38:1055–1061. [ Google Scholar ]
- 88. Lee X.J., YeeLee L., Gan S., Thangalazhy-Gopakumar S., KiatNg H. Biochar potential evaluation of palm oil wastes through slow pyrolysis: thermochemical characterization and pyrolytic kinetic studies. Bioresour. Technol. 2017;236:155–163. doi: 10.1016/j.biortech.2017.03.105. [ DOI ] [ PubMed ] [ Google Scholar ]
- 89. Schwab A.W., Dykstra G.J., Selke E., Sorenson S.C., Pryde E.H. Diesel fuel from thermal decomposition of soybean oil. JAOCS. 1988;65(11):1781–1786. [ Google Scholar ]
- 90. Lua A.C. A detailed study of pyrolysis conditions on the production of steam- activated carbon derived from oil-palm shell and its application in phenol adsorption. Biomass Convers. Biorefinery. 2019:601. doi: 10.1007/s13399-019-00447-9. [ DOI ] [ Google Scholar ]
- 91. He X., Liu Z., Niu X., Li Y., Zhou T., Di Q., Niu Z., Yuan Q. Effects of pyrolysis temperature on the physicochemical properties of gas and biochar obtained from pyrolysis of crop residues. Energy. 2018;143:746–756. [ Google Scholar ]
- 92. Luo G., Chandler D.S., Anjos L.C.A., Eng Ryan J., Jia Pei, Resende F.L.P. Pyrolysis of whole wood chips and rods in a novel ablative reactor. Fuel. 2017;194:229–238. [ Google Scholar ]
- 93. Lui H., Niu S., Lu C. Pyrolysis characteristics of Castor oil through thermogravimetric coupled with fourier transform infrared spectroscopy. Procedia Eng. 2017;205:3705–3710. [ Google Scholar ]
- 94. Alper K., Tekin K., Karagöz S. Pyrolysis of agricultural residues for bio-oil production. Clean Technol. Environ. Policy. 2014;17 doi: 10.1007/s10098-014-0778-8. [ DOI ] [ Google Scholar ]
- 95. Eloka Eboka A.C., Inambao F.L. Effects of CO2 sequestration on lipid and biomass productivity in microalgal biomass production. Appl. Energy. 2017;195(1):1100–1111. [ Google Scholar ]
- 96. Mathimani T., Baldinelli A., Rajendran K., Prabakar D., Matheswaran M. Richard Pieter van Leeuwen, Arivalagan Pugazhendhi.REview on cultivation and thermochemical conversion of microalgae to fuels and chemicals: process evaluation and knowledge gaps. J. Clean. Prod. 2019;208:1053–1064. [ Google Scholar ]
- 97. Pourkarimi S., Hallajisani A., Alizadehdakhel A. Amideddin Nouralishahi Biofuel production through micro- and macroalgae pyrolysis – a review of pyrolysis methods and process parameters. J. Anal. Appl. Pyrolysis. 2019;615(142):104599. [ Google Scholar ]
- 98. Jia L., Cao C., Cheng Z., Wang J., Tsaousis J.H., Yang J., Pan Y., Minggao X., Wang Y. Ex situ catalytic pyrolysis of algal biomass in a double microfixed-bed reactor: catalyst deactivation and its coking behavior. Energy Fuels. 2020;34(2):1918–1928. [ Google Scholar ]
- 99. Shahid A., Ishfaq M., Ahmad M.S., Malik S., Farooq M., Batawi Z.H.A., Shafi M.E., Aloqbi A.A., Gull M., Mehmood M.A. Bioenergy potential of the residual microalgal biomass produced in city wastewater assessed through pyrolysis, kinetics and thermodynamics study to design algal biorefinery. Bioresour. Technol. 2019;289(121701) doi: 10.1016/j.biortech.2019.121701. [ DOI ] [ PubMed ] [ Google Scholar ]
- 100. Vasudev V., Ku X., Lin J. Kinetic study and pyrolysis characteristics of algal and lignocellulosic biomasses. Bioresour. Technol. 2019;288(121496) doi: 10.1016/j.biortech.2019.121496. [ DOI ] [ PubMed ] [ Google Scholar ]
- 101. Yanik J., Stahl R., Troeger N., Sinag A. Pyrolysis of algal biomass. J. Anal. Appl. Pyrolysis. 2013;103:134–141. doi: 10.1016/j.jaap.2012.08.016. [ DOI ] [ Google Scholar ]
- 102. Vargas e Silva F., Monteggia L.O. Pyrolysis of algal biomass obtained from high-rate algae ponds applied to wastewater treatment. Front. Energy Res. 2015;3(31) [ Google Scholar ]
- 103. Adamczyk M., Sajdak M. Pyrolysis behaviours of microalgae nannochloropsis gaditana. Waste Biomass Valorization. 2017:1–15. [ Google Scholar ]
- 104. Trinh N.T., Jensen P.A., Sørensen H.R., Dam-Johansen K., Hvilsted S. Abstract from European Biomass Conference and Exhibition. DTU orbit; 2012. Flash pyrolysis properties of algae and lignin residue. [ Google Scholar ]
- 105. Li Y., Zhou W., Hu B., Min M., Chen P., Ruan R.R. Effect of light intensity on algal biomass accumulation and biodiesel production for mixotrophic strains Chlorella kessleri and Chlorella protothecoide cultivated in highly concentrated municipal wastewater. Biotechnol. Bioeng. 2012;109(9):2222–2229. doi: 10.1002/bit.24491. [ DOI ] [ PubMed ] [ Google Scholar ]
- 106. Li F., Srivatsa S.C., Bhattacharya S. A review on catalytic pyrolysis of microalgae to high- quality bio-oil with low oxygeneous and nitrogenous compounds. Renew. Sustain. Energy 643Rev. 2019;108:481–497. [ Google Scholar ]
- 107. Miao X., Wu Q., Yang C. Fast pyrolysis of microalgae to produce renewable fuels. J. Anal. Appl. Pyrolysis. 2004;71(2):855–863. [ Google Scholar ]
- 108. Zou S., Wu Y., Yang M., Li C., Tong J. Bio-oil production from sub- and supercritical water liquefaction of microalgae Dunaliella tertiolecta and related properties. Energy Environ. Sci. 2010;3:1073–1078. [ Google Scholar ]
- 109. Gao L., Sun J., Wei X., Xiao G. Catalytic pyrolysis of natural algae over Mg-Al layered double oxides/ZSM-5 (MgAl-LDO/ZSM-5) for producing bio-oil with low nitrogen content. Bioresour. Technol. 2017;225:293–298. doi: 10.1016/j.biortech.2016.11.077. [ DOI ] [ PubMed ] [ Google Scholar ]
- 110. Sotoudehniakarani F., Alayat A., McDonald A. Characterization and comparison of pyrolysis products from fast pyrolysis of commercial Chlorella vulgaris and cultivated microalgae. J. Anal. Appl. Pyrolysis. 2019;139:258–273. [ Google Scholar ]
- 111. Ansah E., Wang L., Zhang B., Abolghasem S. Catalytic pyrolysis of raw and hydrothermally carbonized Chlamydomonas debaryana microalgae for denitrogenation and production of aromatic hydrocarbons. Fuel. 2018;228:234–242. [ Google Scholar ]
- 112. Aramkitphotha S., Tanatavikorn H., Yenyuak C., Vitidsant T. Low sulfur fuel oil from blends of microalgae pyrolysis oil and used lubricating oil: properties and economic evaluation. Sustain. Energy Technol. Assess. 2019;31:339–346. [ Google Scholar ]
- 113. Zainan N.H., Srivatsa S.C., Li F., Bhattacharya S. Quality of bio-oil from catalytic pyrolysis of microalgae Chlorella vulgaris. Fuel. 2018;223:12–19. [ Google Scholar ]
- 114. Choi J.H. Pyrolysis of seaweeds for bio-oil and bio-char production. Chem. Eng. Trans. 2014;37:121–126. [ Google Scholar ]
- 115. Gautam R., Vinu R. Non-catalytic fast pyrolysis and catalytic fast pyrolysis of Nannochloropsis oculata using Co-Mo/γ-Al2O3 catalyst for valuable chemicals. Algal Res. 2018;34:12–24. [ Google Scholar ]
- 116. Choi J.H. Pyrolysis of seaweeds for bio-oil and bio-char production. Chem. Eng. Trans. 2014;37:121–126. [ Google Scholar ]
- 117. Ceylan S., Kazan D. Pyrolysis kinetics and thermal characteristics of microalgae Nannochloropsis oculata and Tetraselmis sp. Bioresour. Technol. 2015;(187):1–5. doi: 10.1016/j.biortech.2015.03.081. [ DOI ] [ PubMed ] [ Google Scholar ]
- 118. Gong X., Zhang B., Zhang Y., Huang Y., Xu M. Investigation on pyrolysis of low lipid microalgae Chlorella vulgaris and Dunaliella salina. Energy Fuels. 2014;28(1):95–103. [ Google Scholar ]
- 119. Changwei H., Yang W., Li Y., Dong L., Zhu L., Tong D., Qing R., Fan Y. The direct pyrolysis and catalytic pyrolysis of Nannochloropsis sp. Residue for renewable bio-oils. Bioresour. Technol. 2010;101(12):4593–4599. doi: 10.1016/j.biortech.2010.01.070. [ DOI ] [ PubMed ] [ Google Scholar ]
- 120. Ma C., Geng J., Zhang D., Ning X. Non-catalytic and catalytic pyrolysis of Ulva prolifera macroalgae for production of quality bio-oil. J. Energy Inst. 2020;93(1):303–311. [ Google Scholar ]
- 121. Abd Rahman N.A., Fermoso J., Sanna A. Stability of Li-LSX zeolite in the catalytic pyrolysis of non-treated and acid pre-treated Isochrysis sp. Microalgae. Energies. 2020;13(4):959. [ Google Scholar ]
- 122. Ly H.V., Choi J.H., Woo H.C., Kim S.S., Kim J. Upgrading bio-oil by catalytic fast pyrolysis of acid-washed Saccharina japonica alga in a fluidized-bed reactor. Renew. Energy. 2019;133:11–22. [ Google Scholar ]
- 123. Jafarian S., Tavasoli A. A comparative study on the quality of bioproducts derived from catalytic pyrolysis of green microalgae Spirulina (Arthrospira) plantensis over transition metals supported on HMS-ZSM5 composite. Int. J. Hydrogen Energy. 2018;43(43):19902–19917. [ Google Scholar ]
- 124. Bi Z., Zhang J., Zhu Z., Liang Y., Wiltowski T. Generating biocrude from partially defatted Cryptococcus curvatus yeast residues through catalytic hydrothermal liquefaction. Appl. Energy. 2018;209:435–444. [ Google Scholar ]
- 125. Hidalgo J.M., Horaček J., Matoušek L. Catalytic hydrocracking of vacuum residue and waste cooking oil mixtures. Monatsh. Chem. 2018;149:1167–1177. [ Google Scholar ]
- 126. Thangalazhy-Gopakumar S., Ayalur Chattanathan S., Adhikari S., Gupta R.B. Catalytic pyrolysis of green algae for hydrocarbon production using H(+)ZSM-5 catalyst. Bioresour. Technol. 2012;118:150–157. doi: 10.1016/j.biortech.2012.05.080. [ DOI ] [ PubMed ] [ Google Scholar ]
- 127. Santillan-Jimenez E., Robert P., Tonya M., Craig B., Daniel S., Angelos L., Mark C. Co-processing of hydrothermal liquefaction algal bio-oil and petroleum feedstock to fuel-like hydrocarbons via fluid catalytic cracking. Fuel Process. Technol. 2019;188:164–171. [ Google Scholar ]
- 128. Murata K., Liu Y., Watanabe M.M., Inaba M., Takahara I. Hydrocracking of algae oil into aviation fuel-range hydrocarbons using a Pt–Re catalyst. Energy Fuels. 2014;28(11):6999–7006. [ Google Scholar ]
- 129. Zhao C., Brück T., Lercher J.A. Catalytic deoxygenation of microalgae oil to green hydrocarbons. Green Chem. 2013;15:1720–1739. [ Google Scholar ]
- 130. Woolf D., Lehmann J., Joseph S.C., Christo C., F L Angenent. An open-source biomass pyrolysis reactor: an open source biomass pyrolysis reactor. Biofuels Bioprod. Biorefining. 2017 [ Google Scholar ]
- 131. Mohammed I.Y., Abakr Y.A., Mokaya R. Catalytic upgrading of pyrolytic oil via in-situ hydrodeoxygenation. Waste Biomass Valor. 2020;11:2935–2947. [ Google Scholar ]
- 132. Kumar S. Catalytic approach for production of hydrocarbon rich bio-oil from a Red seaweed species. In: Kumar N., editor. Biotechnology for Biofuels: A Sustainable Green Energy Solution. Springer nature; 2020. [ Google Scholar ]
- 133. Chen X., Chen Y., Yang H., Wang X., Che Q., Chen W., Chen H. Catalytic fast pyrolysis of biomass: selective deoxygenation to balance the quality and yield of bio-oil. Bioresour. Technol. 2019;273:153–158. doi: 10.1016/j.biortech.2018.11.008. [ DOI ] [ PubMed ] [ Google Scholar ]
- View on publisher site
- PDF (2.2 MB)
- Collections
Similar articles
Cited by other articles, links to ncbi databases.
- Download .nbib .nbib
- Format: AMA APA MLA NLM
Add to Collections

IMAGES