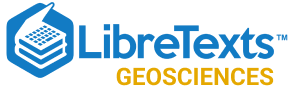
- school Campus Bookshelves
- menu_book Bookshelves
- perm_media Learning Objects
- login Login
- how_to_reg Request Instructor Account
- hub Instructor Commons

Margin Size
- Download Page (PDF)
- Download Full Book (PDF)
- Periodic Table
- Physics Constants
- Scientific Calculator
- Reference & Cite
- Tools expand_more
- Readability
selected template will load here
This action is not available.
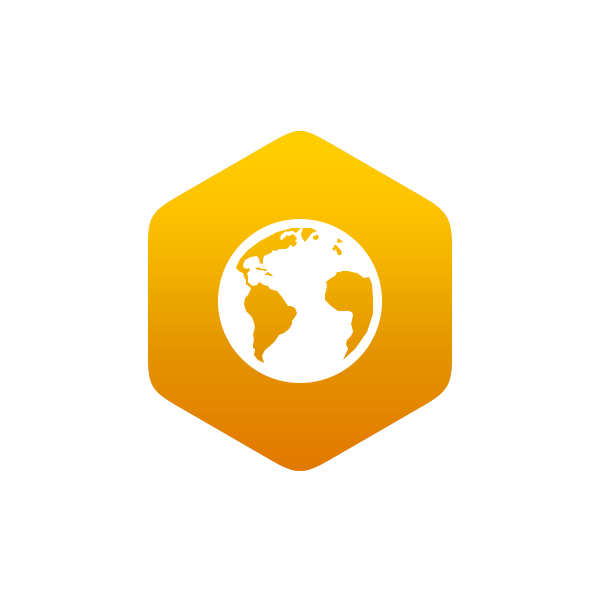
10.4: Plate, Plate Motions, and Plate Boundary Processes
- Last updated
- Save as PDF
- Page ID 7834
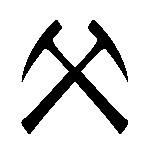
- Steven Earle
- Vancover Island University via BCCampus
\( \newcommand{\vecs}[1]{\overset { \scriptstyle \rightharpoonup} {\mathbf{#1}} } \)
\( \newcommand{\vecd}[1]{\overset{-\!-\!\rightharpoonup}{\vphantom{a}\smash {#1}}} \)
\( \newcommand{\id}{\mathrm{id}}\) \( \newcommand{\Span}{\mathrm{span}}\)
( \newcommand{\kernel}{\mathrm{null}\,}\) \( \newcommand{\range}{\mathrm{range}\,}\)
\( \newcommand{\RealPart}{\mathrm{Re}}\) \( \newcommand{\ImaginaryPart}{\mathrm{Im}}\)
\( \newcommand{\Argument}{\mathrm{Arg}}\) \( \newcommand{\norm}[1]{\| #1 \|}\)
\( \newcommand{\inner}[2]{\langle #1, #2 \rangle}\)
\( \newcommand{\Span}{\mathrm{span}}\)
\( \newcommand{\id}{\mathrm{id}}\)
\( \newcommand{\kernel}{\mathrm{null}\,}\)
\( \newcommand{\range}{\mathrm{range}\,}\)
\( \newcommand{\RealPart}{\mathrm{Re}}\)
\( \newcommand{\ImaginaryPart}{\mathrm{Im}}\)
\( \newcommand{\Argument}{\mathrm{Arg}}\)
\( \newcommand{\norm}[1]{\| #1 \|}\)
\( \newcommand{\Span}{\mathrm{span}}\) \( \newcommand{\AA}{\unicode[.8,0]{x212B}}\)
\( \newcommand{\vectorA}[1]{\vec{#1}} % arrow\)
\( \newcommand{\vectorAt}[1]{\vec{\text{#1}}} % arrow\)
\( \newcommand{\vectorB}[1]{\overset { \scriptstyle \rightharpoonup} {\mathbf{#1}} } \)
\( \newcommand{\vectorC}[1]{\textbf{#1}} \)
\( \newcommand{\vectorD}[1]{\overrightarrow{#1}} \)
\( \newcommand{\vectorDt}[1]{\overrightarrow{\text{#1}}} \)
\( \newcommand{\vectE}[1]{\overset{-\!-\!\rightharpoonup}{\vphantom{a}\smash{\mathbf {#1}}}} \)
Continental drift and sea-floor spreading became widely accepted around 1965 as more and more geologists started thinking in these terms. By the end of 1967 the Earth’s surface had been mapped into a series of plates (Figure \(\PageIndex{1}\)). The major plates are Eurasia, Pacific, India, Australia, North America, South America, Africa, and Antarctic. There are also numerous small plates (e.g., Juan de Fuca, Cocos, Nazca, Scotia, Philippine, Caribbean), and many very small plates or sub-plates. For example the Juan de Fuca Plate is actually three separate plates (Gorda, Juan de Fuca, and Explorer) that all move in the same general direction but at slightly different rates.
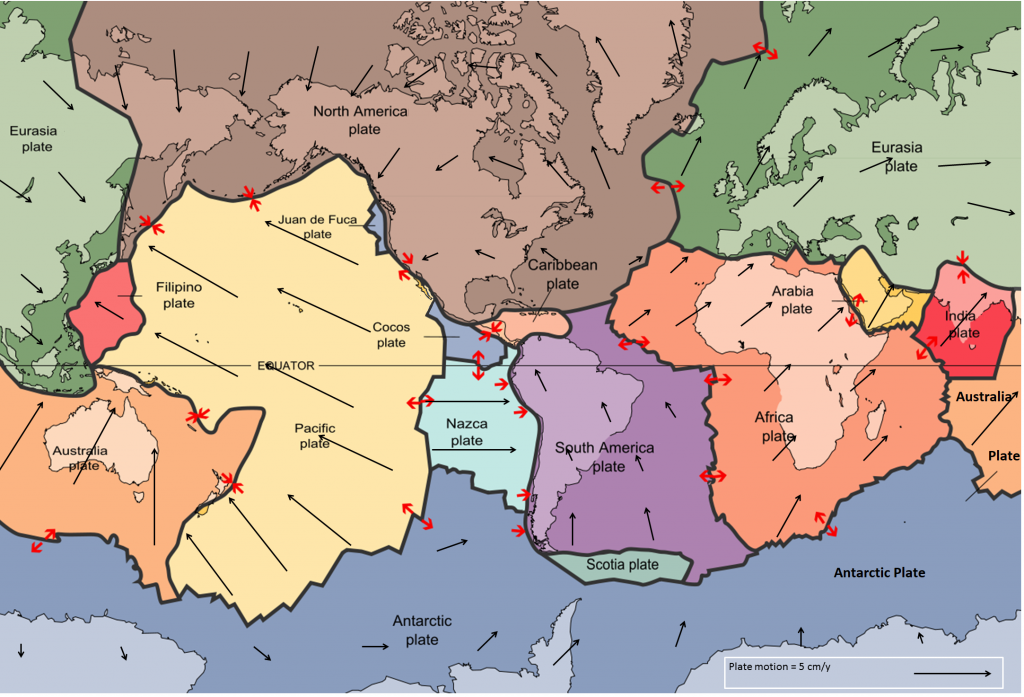
Rates of motions of the major plates range from less than 1 cm/y to over 10 cm/y. The Pacific Plate is the fastest, followed by the Australian and Nazca Plates. The North American Plate is one of the slowest, averaging around 1 cm/y in the south up to almost 4 cm/y in the north.
Plates move as rigid bodies, so it may seem surprising that the North American Plate can be moving at different rates in different places. The explanation is that plates move in a rotational manner. The North American Plate, for example, rotates counter-clockwise; the Eurasian Plate rotates clockwise.
Boundaries between the plates are of three types: divergent (i.e., moving apart) , convergent (i.e., moving together), and transform (moving side by side). Before we talk about processes at plate boundaries, it’s important to point out that there are never gaps between plates. The plates are made up of crust and the lithospheric part of the mantle (Figure \(\PageIndex{2}\)), and even though they are moving all the time, and in different directions, there is never a significant amount of space between them. Plates are thought to move along the lithosphere-asthenosphere boundary, as the asthenosphere is the zone of partial melting. It is assumed that the relative lack of strength of the partial melting zone facilitates the sliding of the lithospheric plates.
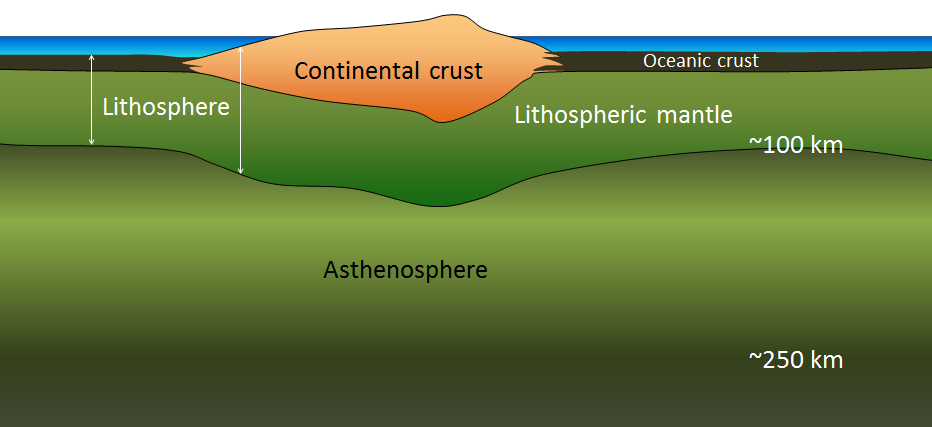
At spreading centres, the lithospheric mantle may be very thin because the upward convective motion of hot mantle material generates temperatures that are too high for the existence of a significant thickness of rigid lithosphere (Figure \(\PageIndex{8}\)). The fact that the plates include both crustal material and lithospheric mantle material makes it possible for a single plate to be made up of both oceanic and continental crust. For example, the North American Plate includes most of North America, plus half of the northern Atlantic Ocean. Similarly the South American Plate extends across the western part of the southern Atlantic Ocean, while the European and African plates each include part of the eastern Atlantic Ocean. The Pacific Plate is almost entirely oceanic, but it does include the part of California west of the San Andreas Fault.
Divergent Boundaries
Divergent boundaries are spreading boundaries, where new oceanic crust is created from magma derived from partial melting of the mantle caused by decompression as hot mantle rock from depth is moved toward the surface (Figure \(\PageIndex{3}\)). The triangular zone of partial melting near the ridge crest is approximately 60 km thick and the proportion of magma is about 10% of the rock volume, thus producing crust that is about 6 km thick. Most divergent boundaries are located at the oceanic ridges (although some are on land), and the crustal material created at a spreading boundary is always oceanic in character; in other words, it is mafic igneous rock (e.g., basalt or gabbro, rich in ferromagnesian minerals). Spreading rates vary considerably, from 2 cm/y to 6 cm/y in the Atlantic, to between 12 cm/y and 20 cm/y in the Pacific. (Note that spreading rates are typically double the velocities of the two plates moving away from a ridge.)
Some of the processes taking place in this setting include:
- Magma from the mantle pushing up to fill the voids left by divergence of the two plates
- Pillow lavas forming where magma is pushed out into seawater (Figure \(\PageIndex{4}\))
- Vertical sheeted dykes intruding into cracks resulting from the spreading
- Magma cooling more slowly in the lower part of the new crust and forming gabbro bodies
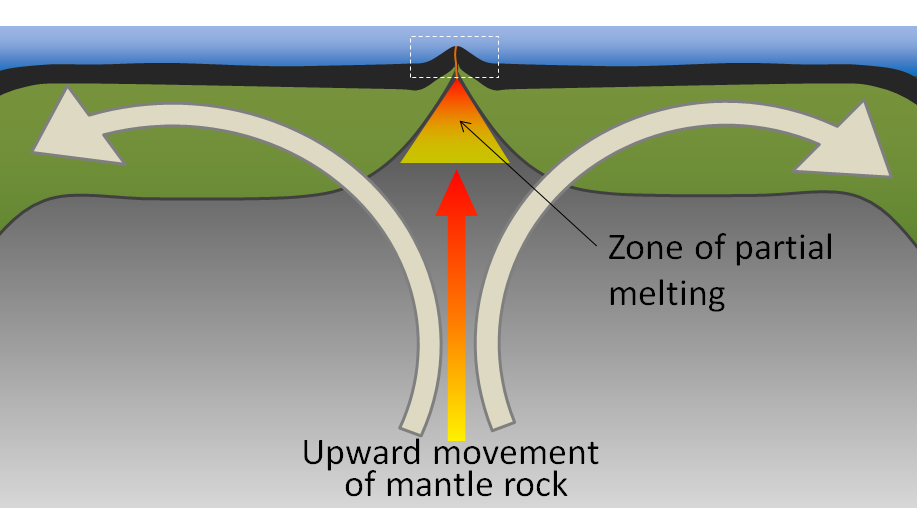
Spreading is hypothesized to start within a continental area with up-warping or doming related to an underlying mantle plume or series of mantle plumes. The buoyancy of the mantle plume material creates a dome within the crust, causing it to fracture in a radial pattern, with three arms spaced at approximately 120° (Figure \(\PageIndex{5}\)). When a series of mantle plumes exists beneath a large continent, the resulting rifts may align and lead to the formation of a rift valley (such as the present-day Great Rift Valley in eastern Africa). It is suggested that this type of valley eventually develops into a linear sea (such as the present-day Red Sea), and finally into an ocean (such as the Atlantic). It is likely that as many as 20 mantle plumes, many of which still exist, were responsible for the initiation of the rifting of Pangea along what is now the mid-Atlantic ridge (see Figure \(\PageIndex{10}\)).
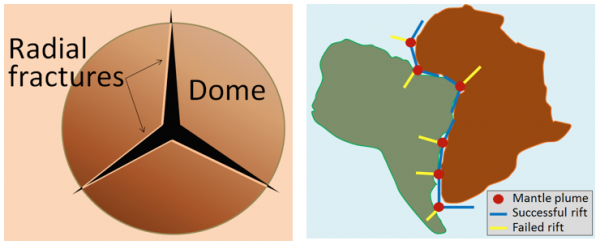
Convergent Boundaries
Convergent boundaries, where two plates are moving toward each other, are of three types, depending on whether oceanic or continental crust is present on either side of the boundary. The types are ocean-ocean, ocean-continent, and continent-continent.
At an ocean-ocean convergent boundary, one of the plates (oceanic crust and lithospheric mantle) is pushed, or subducted , under the other. Often it is the older and colder plate that is denser and subducts beneath the younger and hotter plate. There is commonly an ocean trench along the boundary. The subducted lithosphere descends into the hot mantle at a relatively shallow angle close to the subduction zone, but at steeper angles farther down (up to about 45°). As discussed in the context of subduction-related volcanism in Chapter 4, the significant volume of water within the subducting material is released as the subducting crust is heated. Most of this water is present within the sheet silicate mineral serpentine which is derived from alteration of pyroxene and olivine near the spreading ridge shortly after the rock’s formation. It is released when the oceanic crust is heats and then rises and mixes with the overlying mantle. The addition of water to the hot mantle lowers the rocks’s melting point and leads to the formation of magma (flux melting) (Figure \(\PageIndex{6}\)). The magma, which is lighter than the surrounding mantle material, rises through the mantle and the overlying oceanic crust to the ocean floor where it creates a chain of volcanic islands known as an island arc. A mature island arc develops into a chain of relatively large islands (such as Japan or Indonesia) as more and more volcanic material is extruded and sedimentary rocks accumulate around the islands.
As described above in the context of Benioff zones (Figure \(\PageIndex{6}\)), earthquakes take place close to the boundary between the subducting crust and the overriding crust. The largest earthquakes occur near the surface where the subducting plate is still cold and strong.
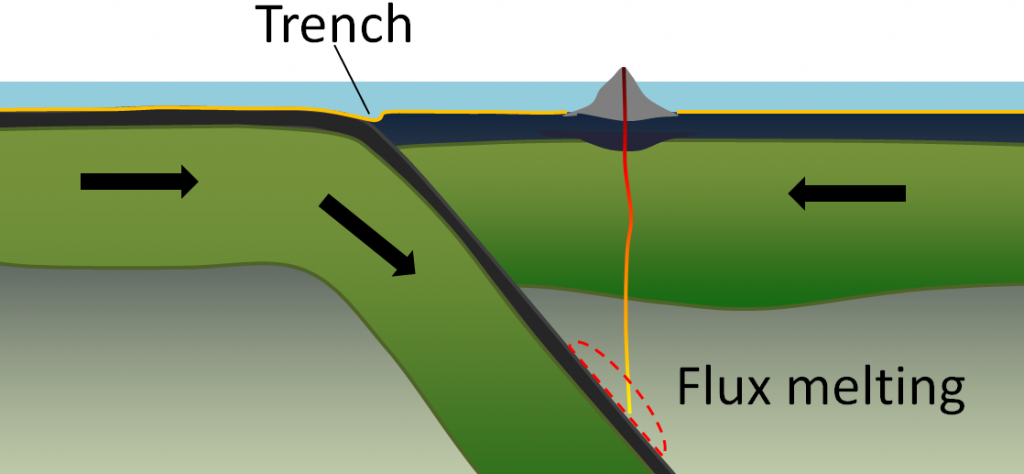
Examples of ocean-ocean convergent zones are subduction of the Pacific Plate beneath the North America Plate south of Alaska (Aleutian Islands) and beneath the Philippine Plate west of the Philippines, subduction of the India Plate beneath the Eurasian Plate south of Indonesia, and subduction of the Atlantic Plate beneath the Caribbean Plate (see Figure \(\PageIndex{1}\)).
At an ocean-continent convergent boundary, the oceanic plate is pushed under the continental plate in the same manner as at an ocean-ocean boundary. Sediment that has accumulated on the continental slope is thrust up into an accretionary wedge, and compression leads to thrusting within the continental plate (Figure \(\PageIndex{7}\)). The mafic magma produced adjacent to the subduction zone rises to the base of the continental crust and leads to partial melting of the crustal rock. The resulting magma ascends through the crust, producing a mountain chain with many volcanoes.
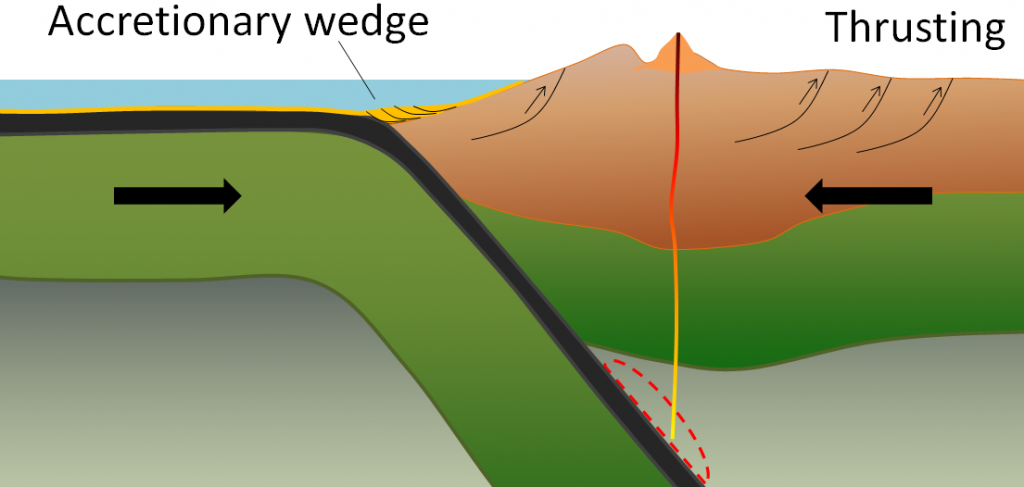
Examples of ocean-continent convergent boundaries are subduction of the Nazca Plate under South America (which has created the Andes Range) and subduction of the Juan de Fuca Plate under North America (creating the mountains Garibaldi, Baker, St. Helens, Rainier, Hood, and Shasta, collectively known as the Cascade Range).
A continent-continent collision occurs when a continent or large island that has been moved along with subducting oceanic crust collides with another continent (Figure \(\PageIndex{8}\)). The colliding continental material will not be subducted because it is too light (i.e., because it is composed largely of light continental rocks [SIAL]), but the root of the oceanic plate will eventually break off and sink into the mantle. There is tremendous deformation of the pre-existing continental rocks, and creation of mountains from that rock, from any sediments that had accumulated along the shores (i.e., within geosynclines) of both continental masses, and commonly also from some ocean crust and upper mantle material.
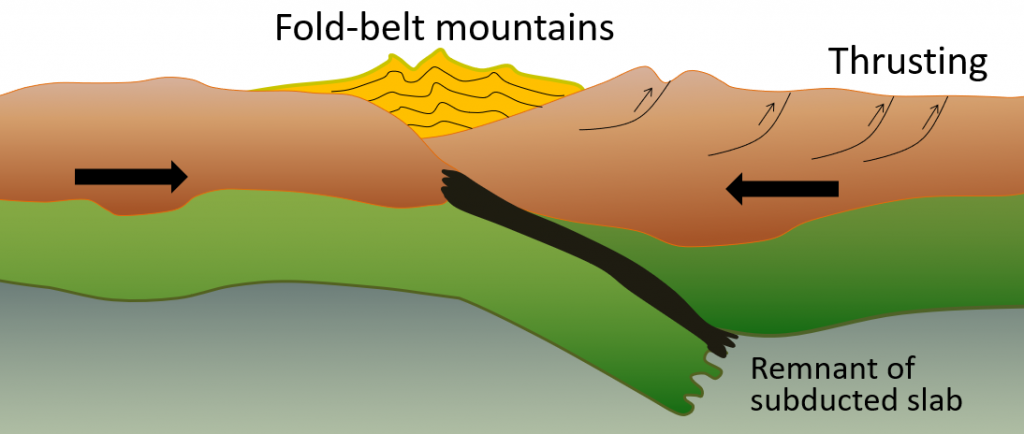
Examples of continent-continent convergent boundaries are the collision of the India Plate with the Eurasian Plate, creating the Himalaya Mountains, and the collision of the African Plate with the Eurasian Plate, creating the series of ranges extending from the Alps in Europe to the Zagros Mountains in Iran. The Rocky Mountains in B.C. and Alberta are also a result of continent-continent collisions.
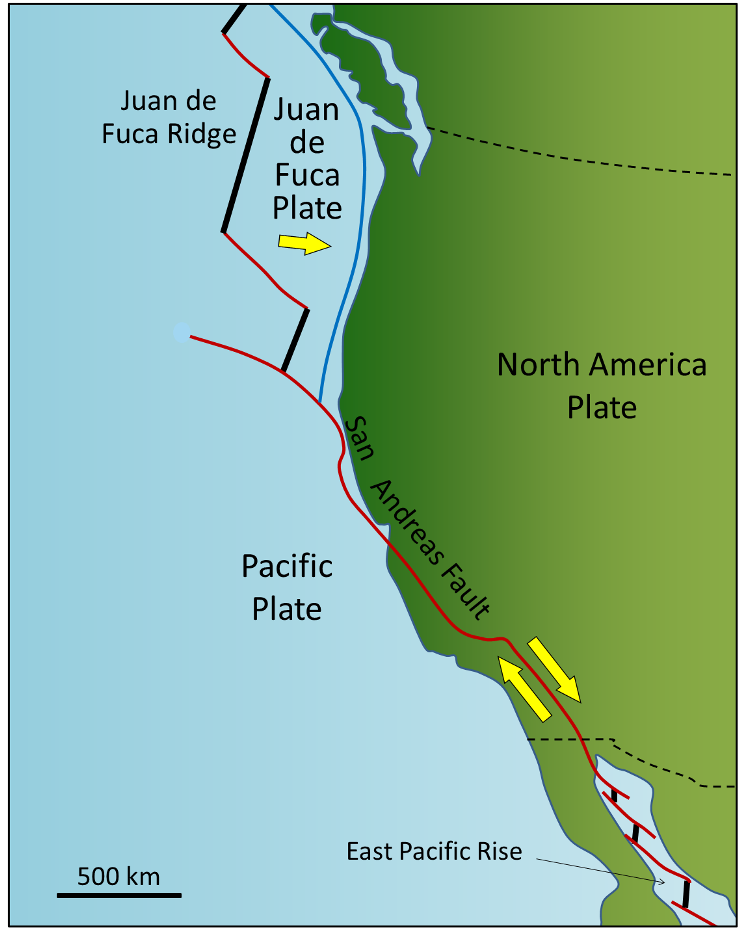
Transform boundaries exist where one plate slides past another without production or destruction of crustal material. As explained above, most transform faults connect segments of mid-ocean ridges and are thus ocean-ocean plate boundaries (Figure \(\PageIndex{11}\)). Some transform faults connect continental parts of plates. An example is the San Andreas Fault, which extends from the southern end of the Juan de Fuca Ridge to the northern end of the East Pacific Rise (ridge) in the Gulf of California (Figures 10.28 and 10.29). The part of California west of the San Andreas Fault and all of Baja California are on the Pacific Plate. Transform faults do not just connect divergent boundaries. For example, the Queen Charlotte Fault connects the north end of the Juan de Fuca Ridge, starting at the north end of Vancouver Island, to the Aleutian subduction zone.
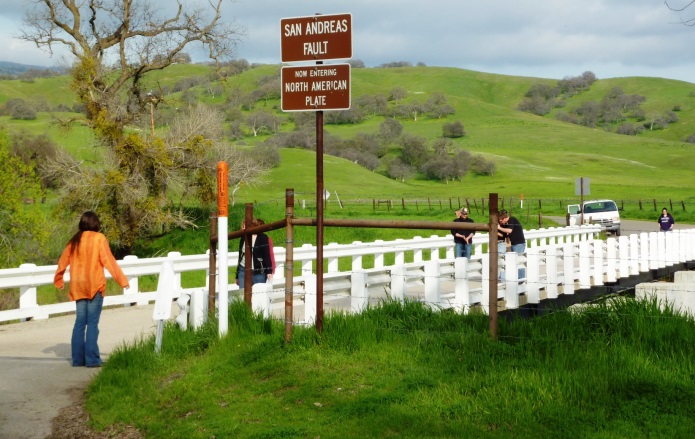
Exercise 10.4 A different type of transform fault
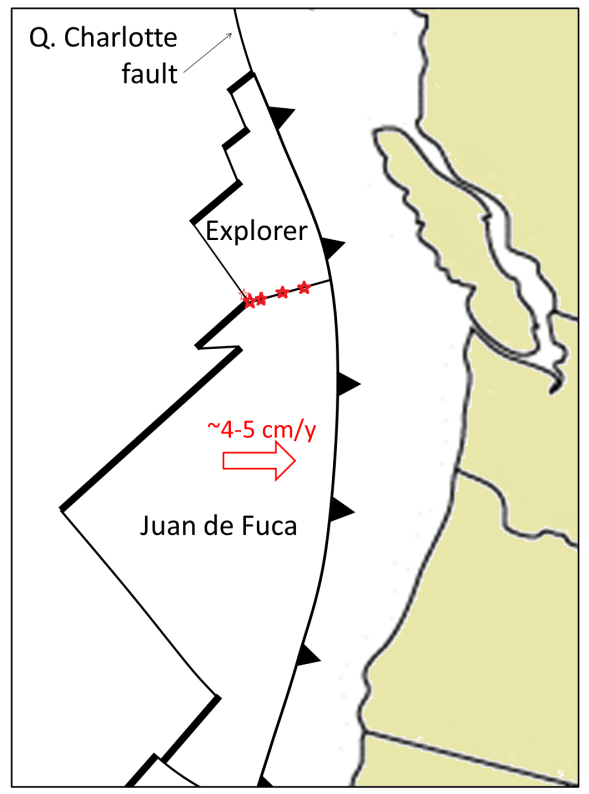
This map shows the Juan de Fuca (JDF) and Explorer Plates off the coast of Vancouver Island. We know that the JDF Plate is moving toward the North American Plate at around 4 centimeters per year to 5 centimeters per year. We think that the Explorer Plate is also moving east, but we don’t know the rate, and there is evidence that it is slower than the JDF Plate.
The boundary between the two plates is the Nootka Fault, which is the location of frequent small-to-medium earthquakes (roughly up to magnitude 5), as depicted by the red stars. Explain why the Nootka Fault is a transform fault, and show the relative sense of motion along the fault with two small arrows.
See Appendix 3 for Exercise 10.4 answers .
As originally described by Wegener in 1915, the present continents were once all part of a supercontinent, which he termed Pangea (meaning all land ). More recent studies of continental matchups and the magnetic ages of ocean-floor rocks have enabled us to reconstruct the history of the break-up of Pangea.
Pangea began to rift apart along a line between Africa and Asia and between North America and South America at around 200 Ma. During the same period, the Atlantic Ocean began to open up between northern Africa and North America, and India broke away from Antarctica. Between 200 and 150 Ma, rifting started between South America and Africa and between North America and Europe, and India moved north toward Asia. By 80 Ma, Africa had separated from South America, most of Europe had separated from North America, and India had separated from Antarctica. By 50 Ma, Australia had separated from Antarctic, and shortly after that, India collided with Asia. To see the timing of these processes for yourself, go to time lapse of Continental Movements .
Within the past few million years, rifting has taken place in the Gulf of Aden and the Red Sea, and also within the Gulf of California. Incipient rifting has begun along the Great Rift Valley of eastern Africa, extending from Ethiopia and Djibouti on the Gulf of Aden (Red Sea) all the way south to Malawi.
Over the next 50 million years, it is likely that there will be full development of the east African rift and creation of new ocean floor. Eventually Africa will split apart. There will also be continued northerly movement of Australia and Indonesia. The western part of California (including Los Angeles and part of San Francisco) will split away from the rest of North America, and eventually sail right by the west coast of Vancouver Island, en route to Alaska. Because the oceanic crust formed by spreading on the mid-Atlantic ridge is not currently being subducted (except in the Caribbean), the Atlantic Ocean is slowly getting bigger, and the Pacific Ocean is getting smaller. If this continues without changing for another couple hundred million years, we will be back to where we started, with one supercontinent.
Pangea, which existed from about 350 to 200 Ma, was not the first supercontinent. It was preceded by Pannotia (600 to 540 Ma), by Rodinia (1,100 to 750 Ma), and by others before that.
In 1966, Tuzo Wilson proposed that there has been a continuous series of cycles of continental rifting and collision; that is, break-up of supercontinents, drifting, collision, and formation of other supercontinents. At present, North and South America, Europe, and Africa are moving with their respective portions of the Atlantic Ocean. The eastern margins of North and South America and the western margins of Europe and Africa are called passive margins because there is no subduction taking place along them.
This situation may not continue for too much longer, however. As the Atlantic Ocean floor gets weighed down around its margins by great thickness of continental sediments (i.e., geosynclines), it will be pushed farther and farther into the mantle, and eventually the oceanic lithosphere may break away from the continental lithosphere (Figure \(\PageIndex{12}\)). A subduction zone will develop, and the oceanic plate will begin to descend under the continent. Once this happens, the continents will no longer continue to move apart because the spreading at the mid-Atlantic ridge will be taken up by subduction. If spreading along the mid-Atlantic ridge continues to be slower than spreading within the Pacific Ocean, the Atlantic Ocean will start to close up, and eventually (in a 100 million years or more) North and South America will collide with Europe and Africa.
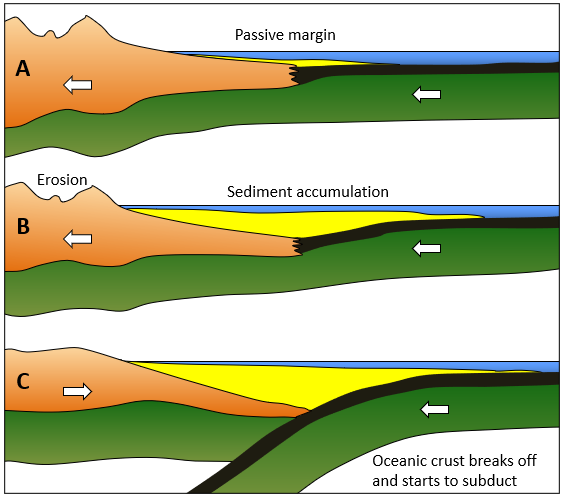
There is strong evidence around the margins of the Atlantic Ocean that this process has taken place before. The roots of ancient mountain belts, which are present along the eastern margin of North America, the western margin of Europe, and the northwestern margin of Africa, show that these land masses once collided with each other to form a mountain chain, possibly as big as the Himalayas. The apparent line of collision runs between Norway and Sweden, between Scotland and England, through Ireland, through Newfoundland, and the Maritimes, through the northeastern and eastern states, and across the northern end of Florida. When rifting of Pangea started at approximately 200 Ma, the fissuring was along a different line from the line of the earlier collision. This is why some of the mountain chains formed during the earlier collision can be traced from Europe to North America and from Europe to Africa.
That the Atlantic Ocean rift may have occurred in approximately the same place during two separate events several hundred million years apart is probably no coincidence. The series of hot spots that has been identified in the Atlantic Ocean may also have existed for several hundred million years, and thus may have contributed to rifting in roughly the same place on at least two separate occasions (Figure \(\PageIndex{13}\)).
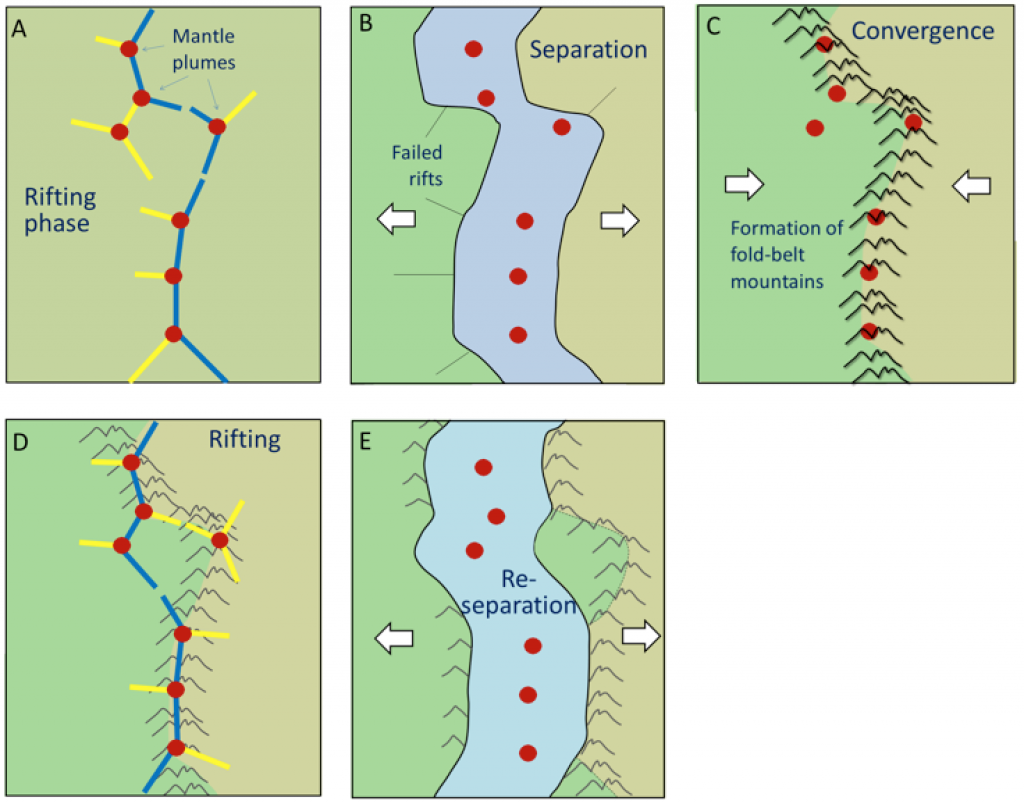
Exercise 10.5 Getting to know the plates and their boundaries
This map shows the boundaries between the major plates. Without referring to the plate map in Figure \(\PageIndex{1}\), or any other resources, write in the names of as many of the plates as you can. Start with the major plates, and then work on the smaller ones. Don’t worry if you can’t name them all.
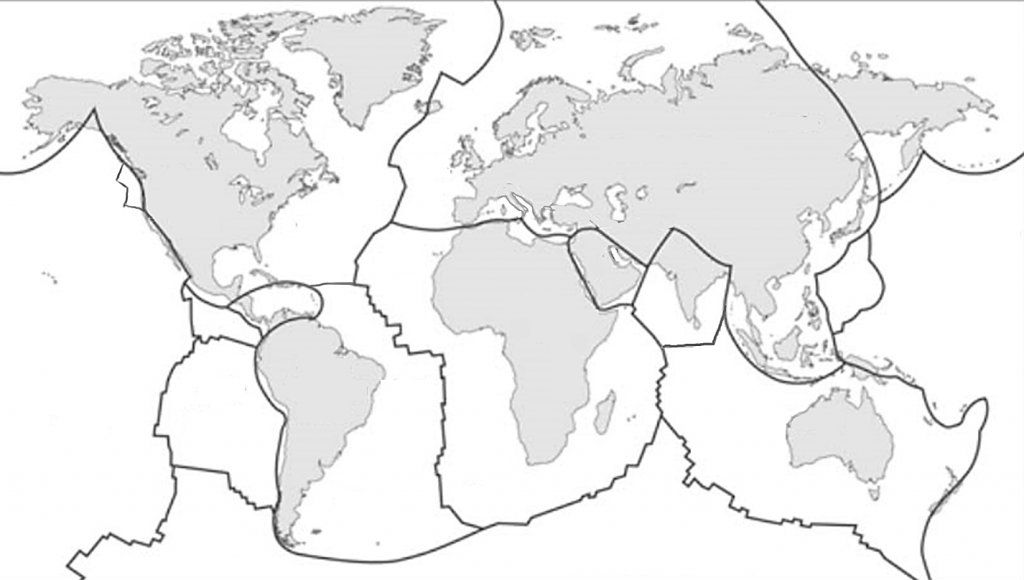
Once you’ve named most of the plates, draw arrows to show the general plate motions. Finally, using a highlighter or colored pencil, label as many of the boundaries as you can as divergent, convergent, or transform.
See Appendix 3 for Exercise 10.5 answers .
Image descriptions
[Return to Figure \(\PageIndex{1}\)]
Media Attributions
- Figure \(\PageIndex{1}\): “Plates tect2 en” by the USGS . Adapted by Steven Earle. Public domain.
- Figures 10.4.2, 10.4.3, 10.4.5, 10.4.6, 10.4.7, 10.4.8, 10.4.9, 10.4.10, 10.4.11, 10.4.12, 10.4.13, 10.4.14: © Steven Earle. CC BY.
- Figure \(\PageIndex{4}\): © Steven Earle. CC BY. Based on Keary and Vine, 1996, Global Tectonics (2ed), Blackwell Science Ltd., Oxford.
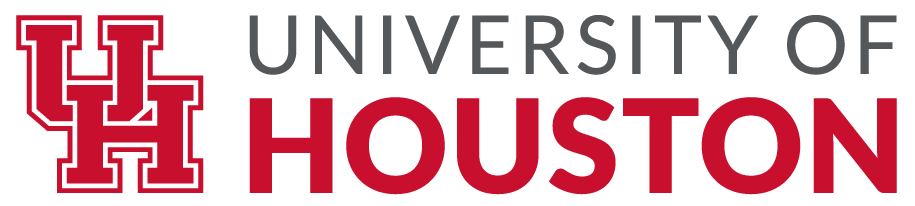
Want to create or adapt books like this? Learn more about how Pressbooks supports open publishing practices.
Chapter 1: Plate Tectonics
The 2 nd edition is now available click here., learning objectives.
The goals of this chapter are to:
- Identify types of plate boundaries and compare their characteristic earthquake and volcanic activities
- Assess the basic lines of evidence supporting plate tectonics
- Explain how ancient plate boundaries affect modern topography
1.1 Introduction
Plate tectonics is the grand unifying theory in geology. It gets that title because many topics in geology can be explained, in some way, by the movement of tectonic plates. Tectonic plates are composed of Earth’s crust and the uppermost, rigid portion of the mantle. Together they are called the lithosphere . Earth’s crust comes in 2 “flavors”: oceanic and continental (Table 1.1).
Lithospheric plates move around the globe in different directions and come in many different shapes and sizes. Their movement rate is millimeters to a few centimeters per year, similar to the rate that your fingernails grow. Motion between tectonic plates can be divergent , convergent , or transform . In divergent boundaries, plates are moving away from each other; in convergent boundaries, plates are moving toward each other; and in transform boundaries, plates are sliding past each other. The type of crust on each plate determines the geologic behavior of the boundary (Figure 1.1).
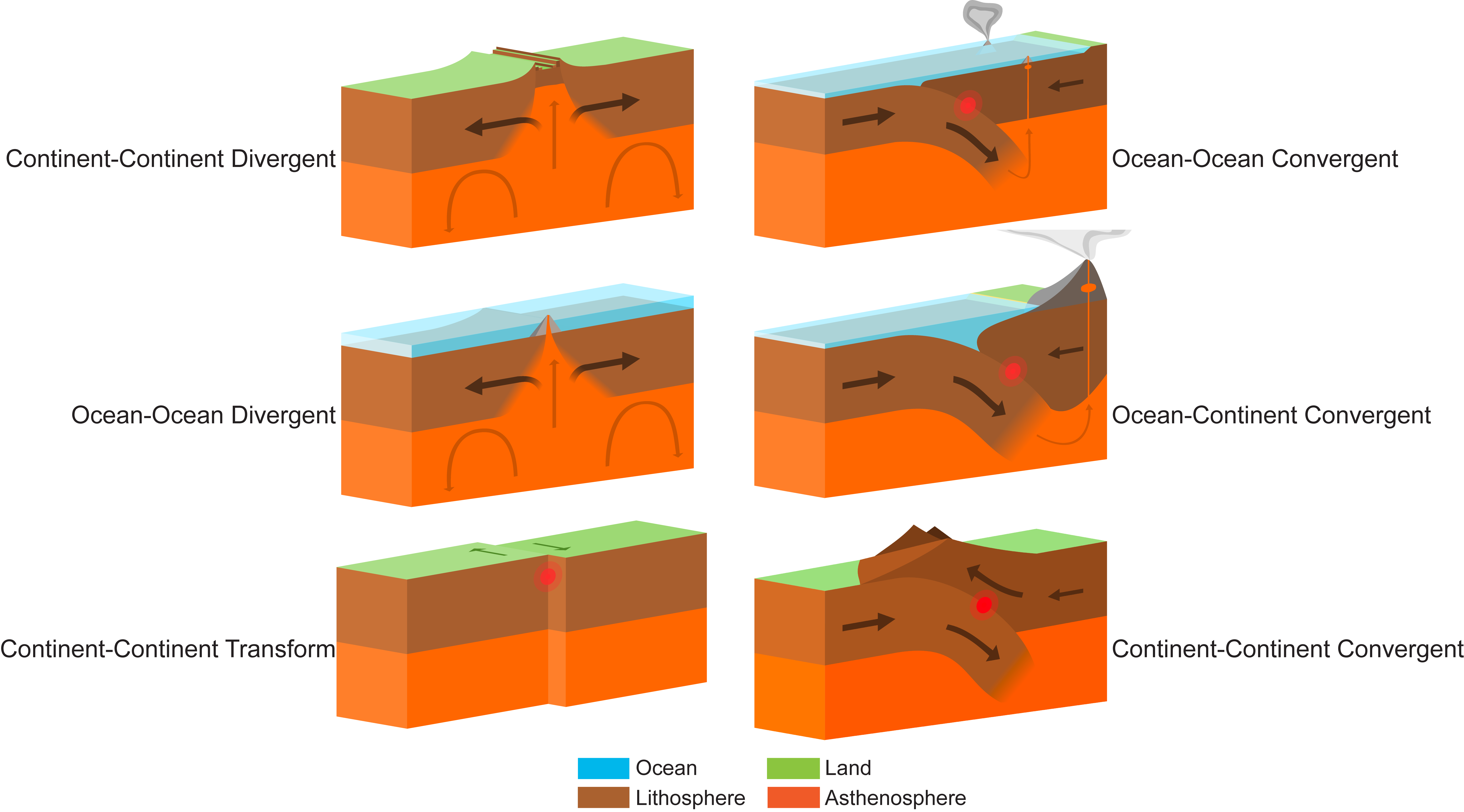
Exercise 1.1 – Reconstructing Positions of Continents Using Wegener’s Evidence
When Alfred Wegener came up with his continental drift hypothesis in the early 1900s, he used several lines of evidence to support his idea. He also proposed that 200 million years ago, all continents were together in a single supercontinent called Pangea. In this exercise, you will use the fit of the continents and matching fossil evidence to piece together Pangea. This exercise is adapted from “ This Dynamic Planet ” by the USGS.
- Label the landmasses of each continent in Figure 1.2.
- Color the fossil areas to match the legend below.
- Cut out each of the continents along the edge of the continental shelf (the outermost dark line).
- Try to logically piece the continents together so that they form a giant supercontinent.
- When you are satisfied with the fit of the continents, discuss the evidence with your classmates and decide if the evidence is compelling or not. Explain your decision and reasoning on the evidence.
- Pangea began to break apart about 200 Ma resulting in the formation of the Atlantic Ocean. Using the map in Figure 1.3, calculate the spreading rate of the Mid-Atlantic Ridge in mm/yr. (Hint: measure the distance from the easternmost tip of South America to the inside curve of western Africa). ____________________
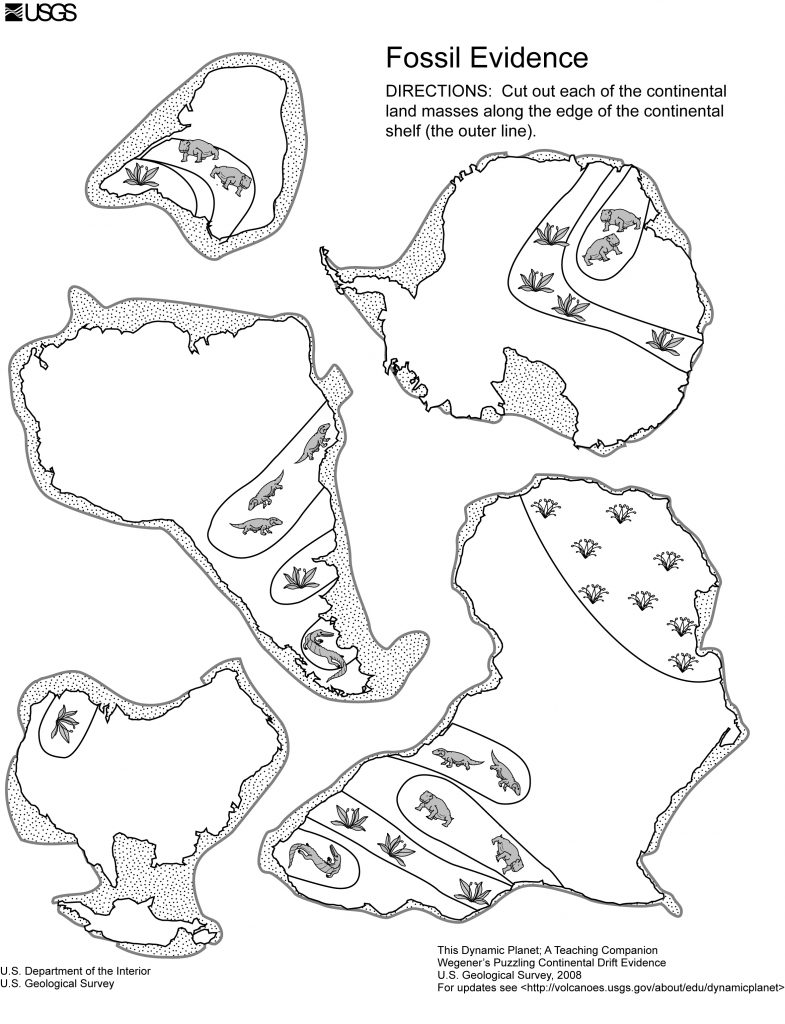
1.2 Plate Tectonics, Earthquakes, and Volcanoes
Plate tectonic boundaries are often associated with earthquakes and volcanic activity. By looking at maps for the distribution of earthquakes and volcanoes worldwide (Figures 1.4-1.5), you can interpret the boundaries between the major tectonic plates. Generally, divergent plate boundaries are characterized by shallow earthquakes and some volcanism. Convergent boundaries have a range of earthquake depths from shallow to deep, and many have volcanoes as a result of subduction . Subduction occurs in convergent boundaries where the denser, oceanic plate descends into the mantle beneath the overriding plate. Convergent boundaries also tend to produce linear and curved mountain belts . Transform boundaries typically have shallow earthquakes and no volcanoes.
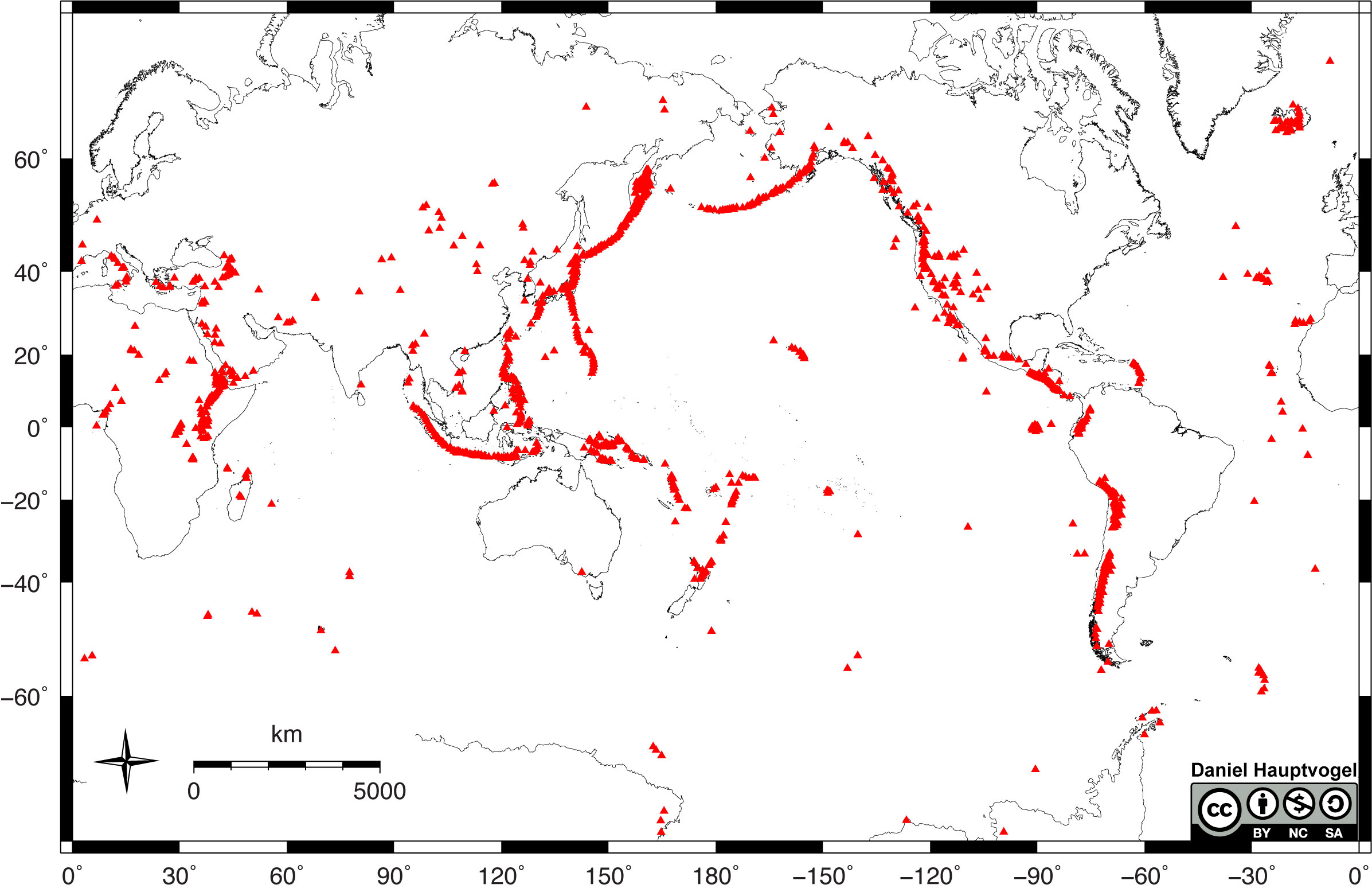
Exercise 1.2 – Modern Examples of Plate Tectonic Boundaries
Each type of plate boundary has distinct earthquake and volcanic patterns. Using observational and critical thinking skills, answer the following questions:
- Observe the patterns amongst the earthquake and volcano location maps (Figures 1.4-1.5). Hypothesize where you think the major plate boundaries exist and draw those boundaries on the blank map in Figure 1.6 using three different colors to identify the type of motion for each boundary (example: red for divergent boundaries, blue for convergent boundaries, and green for transform boundaries).
- Which type of boundary (divergent, convergent, or transform) is the most abundant? ______________________________________
- Continent-Continent Convergence (CCC)
- Ocean-Ocean Convergence (OOC)
- Continent-Ocean Convergence (COC)
- Continent-Continent Divergence (CCD)
- Ocean-Ocean Divergence (OOD)
- Continent-Continent Transform (CCT)
- What type of plate boundary is associated with most of the deep earthquakes?______________________
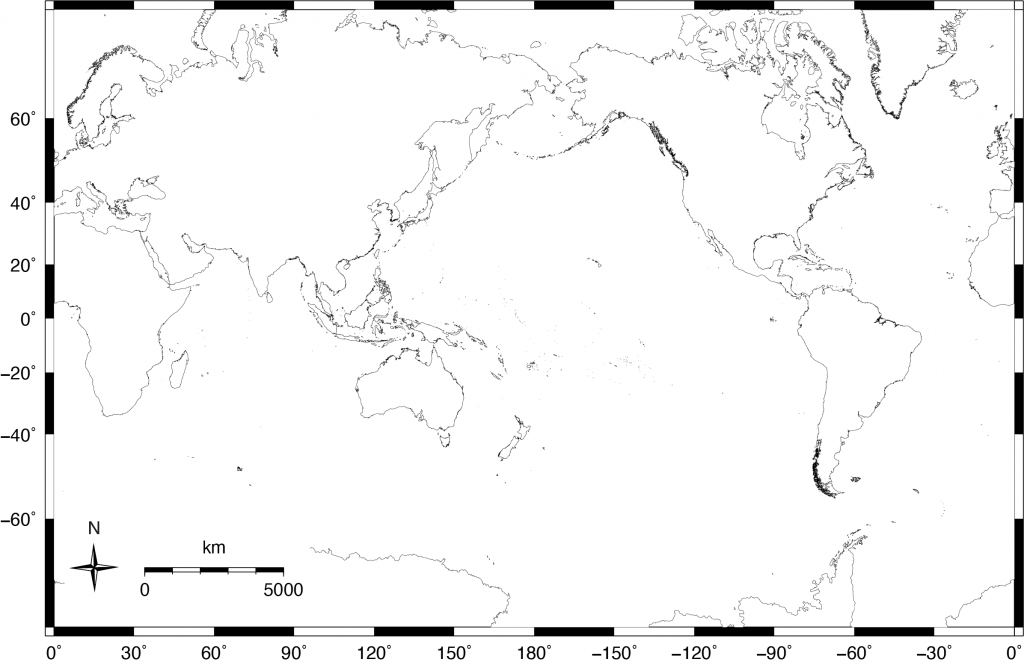
Earthquake locations can tell you more about an area than what type of plate boundary is there. For example, in subduction zones, most earthquakes occur along the boundary between the subducting slab and the overriding slab. The angle of subduction is not always constant and can vary from one boundary to the next and can even vary along the same boundary. When a plate subducts at a low angle, it is called flat-slab subduction. The effects of flat-slab subduction are many, including shallower earthquakes, uplifting of mountains, and the location and activity of volcanoes.
Exercise 1.3 – Interpreting Subduction Angle from Earthquake Data
The Western margin of South America is a tectonically active region where the Nazca Plate subducts under the South American Plate (Figure 1.7), creating the Andes Mountains . Even though the entire coast is part of the same subduction zone, the subduction process doesn’t look the same throughout. Tables 1.3 and 1.4 contain earthquake data from two different locations of the subduction zone, one from central Chile and another near the Chile-Peru border. The location data are the distance each earthquake was from the trench and how deep within the Earth it was.
- Using the graph paper provided by your instructor, plot the distance of the earthquake foci (hypocenters) from the trench on the horizontal axis and the depth of the earthquakes on the vertical axis; the recommended scale is 1 cm = 10 km. Connect the plotted points to create an approximate cross-section of the subduction zone at the two locations.
- Look at the graph you made, which region has a steeper subduction angle, the Chile-Peru border or central Chile? ____________________
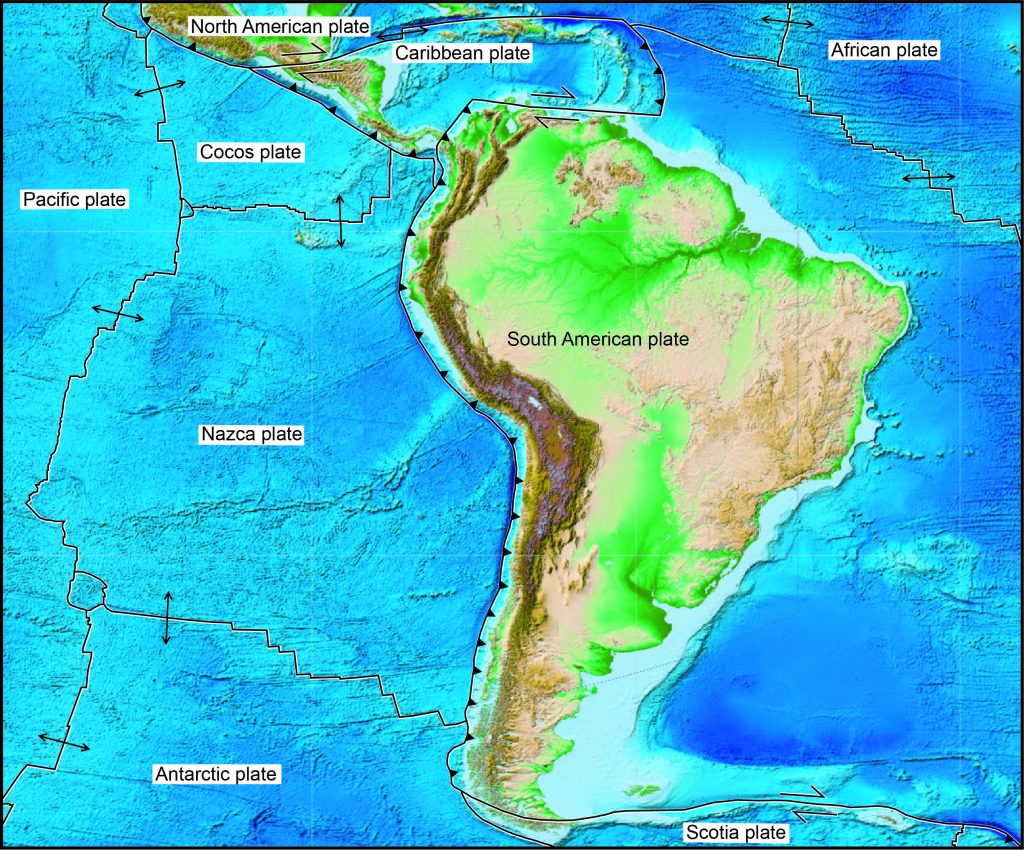
1.3 Plate Tectonics and Topography
Geologists can observe most of the processes occurring at plate tectonic boundaries today (earthquakes, volcanoes, mountain building, etc.). However, understanding the plate tectonic activity of the geologic past is more difficult because the events have already happened. Hence, geologists use processes that occur in the present to interpret processes that occurred in the past. This is known as uniformitarianism . One way geologists can interpret ancient plate tectonic activity is to look at the topography of an area. Topography is the study of shapes and features of the land surface. When studying features on the seafloor, the topography is instead referred to as bathymetry because this data is referencing how deep a feature is. There are numerous ways of looking at the topography of Earth’s surface, including satellite imagery, topographic maps, shaded relief maps, and digital elevation models.
Exercise 1.4 – Interpreting Plate Boundaries from Topographic Profiles
Below are five topographic profiles showing different plate boundary configurations. A topographic profile is a graph that shows elevation changes as you walk from one point on the Earth to another. These are all made with vertical exaggeration (length/elevation) of 50:1. This overemphasizes the changes in topography. In all of these profiles, the 0 value on the vertical axis is at sea level.
- For the topographic profiles in Table 1.4, identify what types of plate boundaries are shown using the names from Figure 1.1. Pay close attention to the y-axis versus x-axis.
- On each profile, draw the location of the boundary between the two plates. You can show this as a single line.
- For each profile, label features such as oceanic and/or continental crust, mid-ocean ridges, volcanoes, mountain belts, and trenches.
- Indicate which direction each tectonic plate is moving (you can use arrows for this).
Geologists can use topography to get a broad sense of the tectonic history of an area. Generally speaking, plate tectonic activity tends to produce elevation changes at or near the plate boundary, especially in convergent settings. The collision of two plates leads to suturing ; the two plates become one when the collision ends. Evidence of these ancient boundaries is most commonly in the form of linear mountain belts that are not currently near a plate tectonic boundary. For example, an eroded, linear mountain belt in the middle of a continent would indicate that area was part of a convergent boundary deep in the geologic past and likely a continent-continent collision. The Ural Mountains in Russia fit this description (Figure 1.8). They formed during an orogeny 240 to 300 million years ago and now serve as the boundary between Europe and Asia.
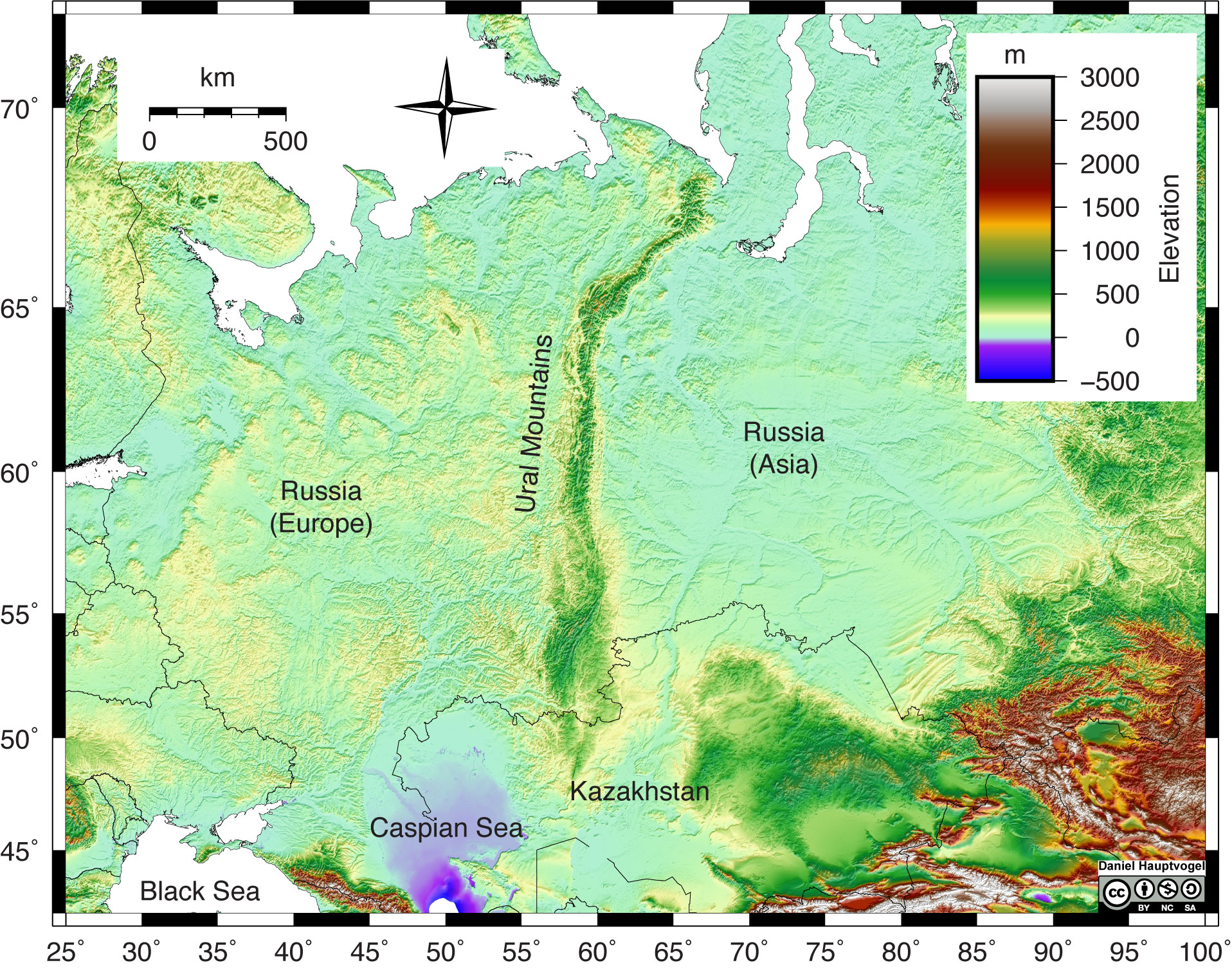
Exercise 1.5 – Interpreting Ancient Plate Tectonic Boundaries
- Look at the topographic map for part of North America (Figure 1.9). Mark two areas that you think have been through major convergence of tectonic plates.

When most people think about tectonic plate boundaries, they often visualize parallel, symmetric lines separating the plates. This is not always the case in the real world as many plate boundaries are curved or segmented; this is because Earth is a sphere. Think about this: if you had a ball and tried to wrap it with a flat sheet of paper, would the paper wrap around it perfectly smooth? The answer is no; the paper will want to fold in some places and tear in other places. The tectonic plates behave similarly to the paper. Of course, other factors affect the shape of a boundary. Evidence of these plate boundaries is also contained in the topography of continents because not all mountain belts are straight lines.
Exercise 1.6 – Ancient Plate Boundaries in Texas, Oklahoma, New Mexico, and Northeastern Mexico
Below is a topographic map of Texas, Oklahoma, New Mexico, and northeastern Mexico (Figure 1.11). This area is not near an active plate tectonic boundary today; the closest boundary is in the Gulf of Mexico. However, there is evidence in this topography to indicate it was part of a plate tectonic boundary at least twice in the geologic past.
- Based on the topography, mark two areas that have been part of a plate tectonic boundary in the geologic past. Topographic changes do not need to be symmetric as some tectonic processes are not symmetric.
- One of these boundaries is older than the other. Label the old and young boundaries.
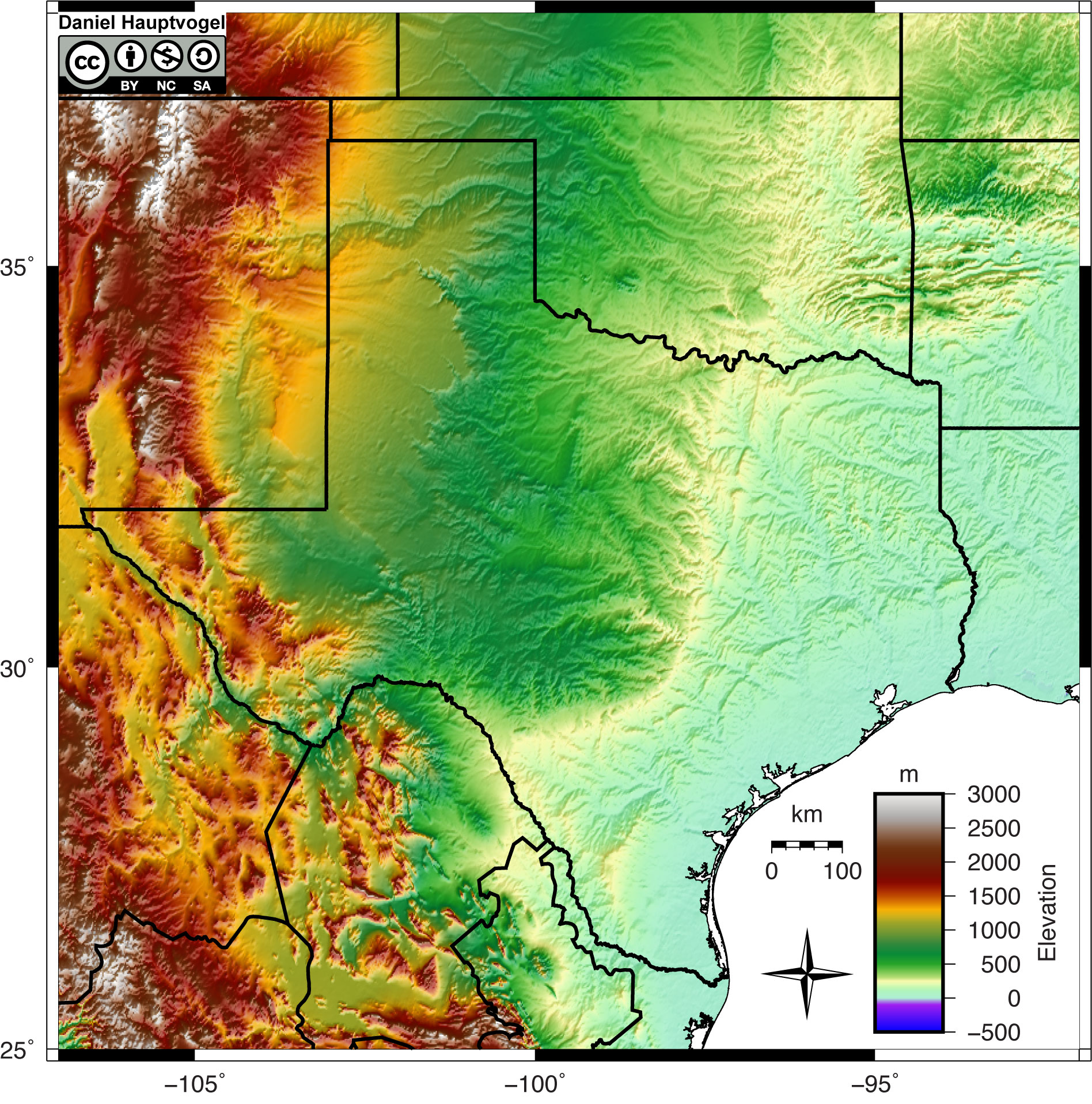
As tectonic plates move, they ride over stationary “ hotspots ” of heat from the mantle . Hotspots are still a poorly understood geologic phenomenon, but they allow extremely hot mantle material to rise close to the surface. Hotspots are marked by volcanoes, which come from melting of the mantle and crust directly above a hotspot. If they occur under oceanic crust, they produce basalts. On the other hand, if they are under continental crust, they form both basalts and rhyolites, often called bimodal volcanism. Under North America, there are two hotspots: The Yellowstone hotspot, which is currently under Yellowstone National Park in Wyoming and Montana, and the Anahim hotspot in central British Columbia, Canada. As the North American Plate moves over these hotspots, calderas form from volcanic activity; one of the largest volcanic eruptions ever occurred when the Gray’s Landing volcanics erupted 8.72 million years ago above the Yellowstone hotspot. One of the controversies is whether the hot spot is still capable of supereruptions or whether the volume of eruptive material is waning.
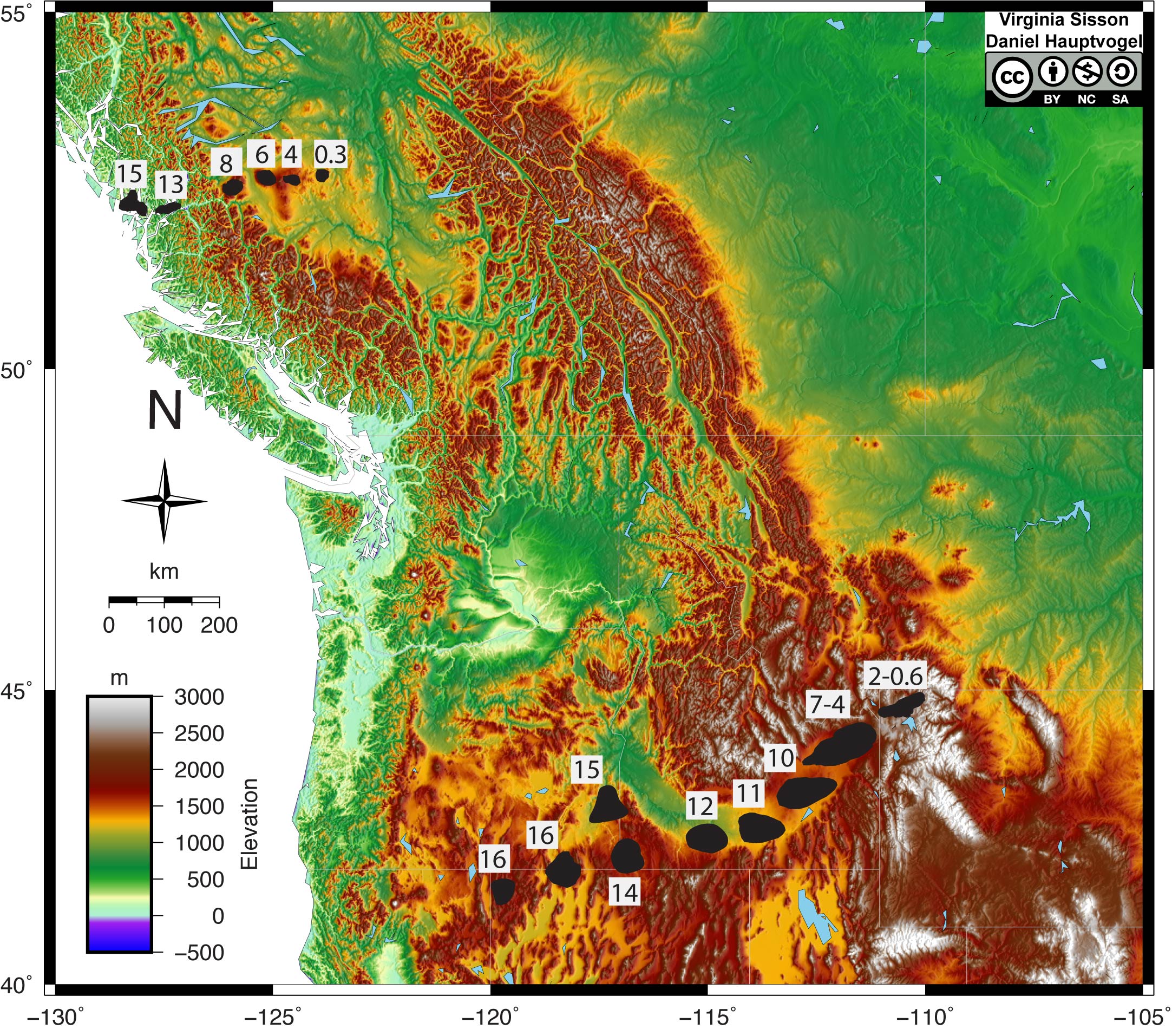
Exercise 1.7 – Tracking North American Hotspots
Use Figure 1.12 to answer the following questions about North American hotspots.
- Angle for Yellowstone: ____________________
- Angle for Anahim: ____________________
- Rate of plate motion for Yellowstone: ____________________
- Rate of plate motion for Anahim: ____________________
- Are these the same for the two hotspots? ____________________
The answer may not be apparent as we don’t often move things around on a sphere. Instead, we think of motion as a straight line from point a to point b. These hotspots are on the North American plate which means the plate rotates around a point in the middle of northern Quebec. Since they rotate around a point on a sphere, different locations on the plate move at dissimilar speeds and directions. Geoscientists call this an Euler pole .
Additional Information
Exercise contributions.
Daniel Hauptvogel, Virginia Sisson, Carlos Andrade, Melissa Hansen
Knott, T.R., Branney, M.J., Reichow, M.K., Finn, D.R., Tapster, S., and Coe, R.S., 2020, Discovery of two new super-eruptions from the Yellowstone hotspot track (USA) Is the Yellowstone hotspot waning? Geology, v. 48, p. 934-938. doi.org/10.1130/G47384.1
Martinod, J., Husson, L., Roperch, P., Guillaume, B., and Espurt, N., 2010, Horizontal subduction zones, convergence velocity and the building of the Andes. Earth and Planetary Science Letters, v. 299, pp. 299-309. DOI:10.1016/j.epsl.2010.09.010 .
Google Earth Locations
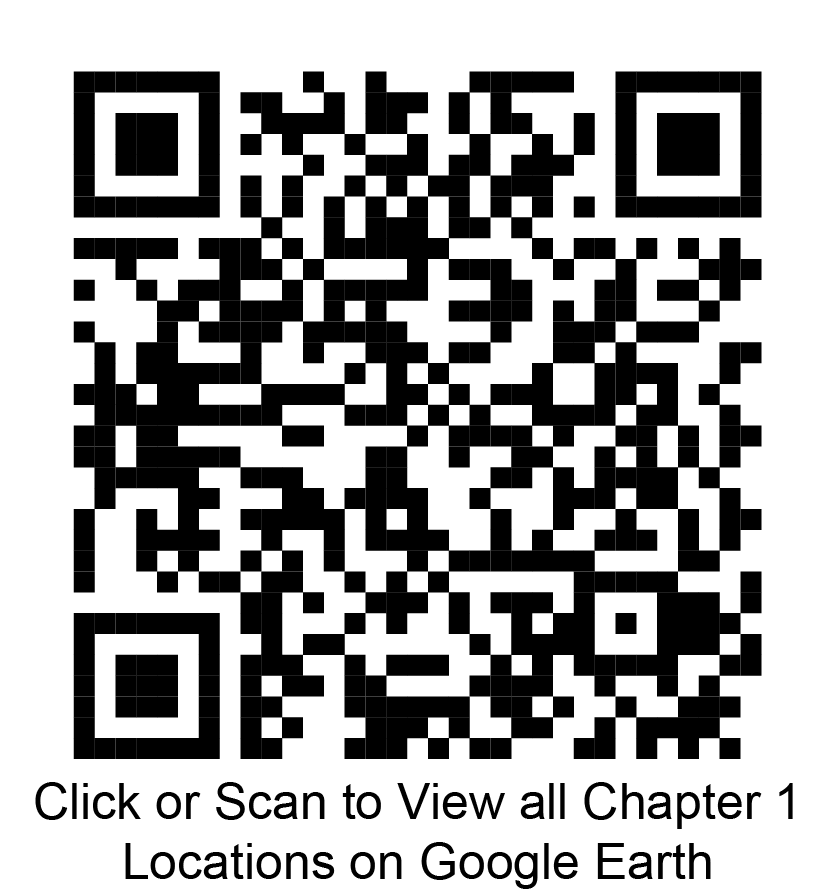
the uppermost layer of the Earth as defined by physical properties. It is composed of the crust and uppermost, rigid part of the mantle.
a linear feature where two plate boundaries move apart from each other
a plate boundary where two plates move towards each other
a plate boundary where two plate boundaries move horizontally beside each other
the hypothesis proposed by Alfred Wegener in 1915 that stated the continents were moving
the climate during some past geological time
an ancient landmass of almost all of the continental crust or supercontinent that formed in the late Paleozoic
a process that occurs at convergent plate boundaries where one plate descends beneath another into Earth's mantle.
an aligned group of mountain ranges that form from the same cause, usually an orogeny or convergent plate boundary
a topographic depression of the sea floor, relatively narrow in width, but very long usually associated with convergent plate boundaries
the theory that the same natural laws and processes that operate in our present-day scientific observations have always operated in the universe in the past and apply everywhere in the universe. It is sometimes referenced as "The present is the key to the past".
the zone where two continental plates meet during convergence
a mountain-building event typically resulting from a convergent tectonic boundary
linear volcanic regions that are probably fed by underlying mantle that is anomalously hot compared with the surrounding mantle
a layer in the Earth or a planet that is between the core and crust
The Story of Earth: An Observational Guide Copyright © by Daniel Hauptvogel & Virginia Sisson is licensed under a Creative Commons Attribution-NonCommercial-ShareAlike 4.0 International License , except where otherwise noted.
Share This Book
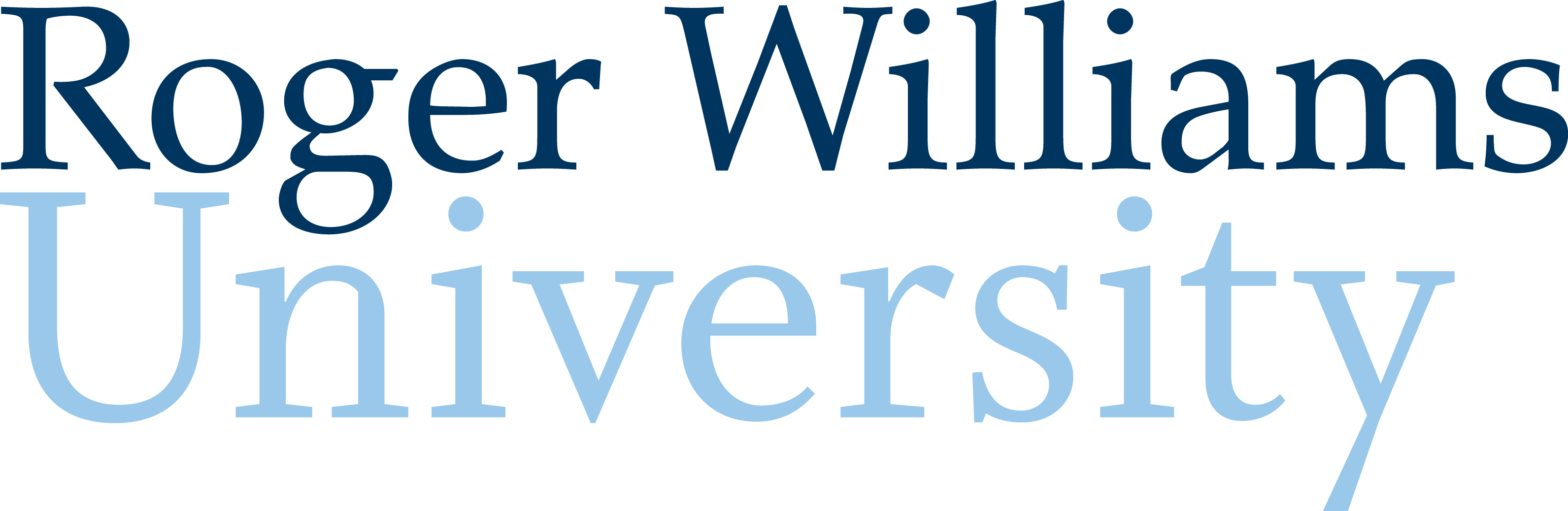
Want to create or adapt books like this? Learn more about how Pressbooks supports open publishing practices.
4.1 Alfred Wegener and the Theory of Plate Tectonics
Modified from "Physical Geology" by Steven Earle*
If you look at a map of Earth, you may notice that some of the continents seem to fit together. An early reference to this phenomenon came from Francis Bacon in the 17th century, who noticed the similarities in the Atlantic coasts of Africa, and North and South America. This apparent fit is due to the fact the continents were once connected, and have since moved apart in what has been called continental drift . However, we now know that it is not just the continents that move, so a more correct term is plate tectonics . We can credit Alfred Wegener (Figure 4.1.1) as the originator of this idea.
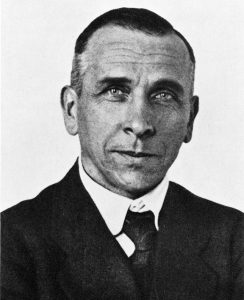
Alfred Wegener (1880-1930) earned a PhD in astronomy at the University of Berlin in 1904, but he had always been interested in geophysics and meteorology and spent most of his academic career working in meteorology. In 1911 he happened on a scientific publication that included a description of the existence of matching Permian -aged terrestrial fossils in various parts of South America, Africa, India, Antarctica, and Australia (Figure 4.1.2). Wegener concluded that this distribution of fossils could only exist if these continents were joined together. Furthermore, some of these transcontinental areas have similar fossils until around 150 million years ago, then they begin to diverge, suggesting that the areas eventually separated and speciation took different paths on the separate continents. Wegener coined the term Pangaea (“all land”) for the supercontinent from which all of the present-day continents diverged.
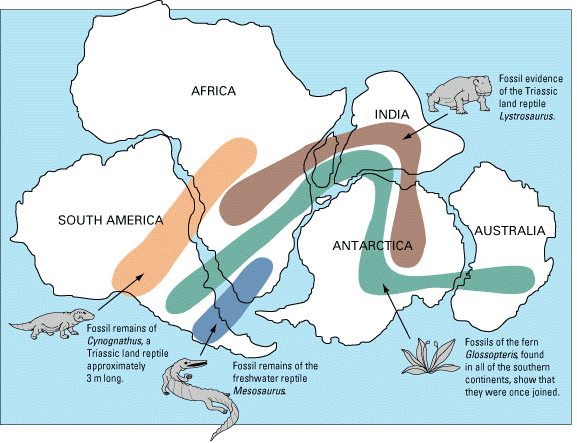
Wegener pursued his theory with determination — combing the libraries, consulting with colleagues, and making observations — looking for evidence to support it. In addition to the fit of the continents and the fossil evidence, Wegener relied heavily on matching geological patterns across oceans, such as sedimentary strata in South America matching those in Africa (Fig. 4.1.3), North American coalfields matching those in Europe, and the mountains of Atlantic Canada matching those of northern Britain both in morphology and rock type.
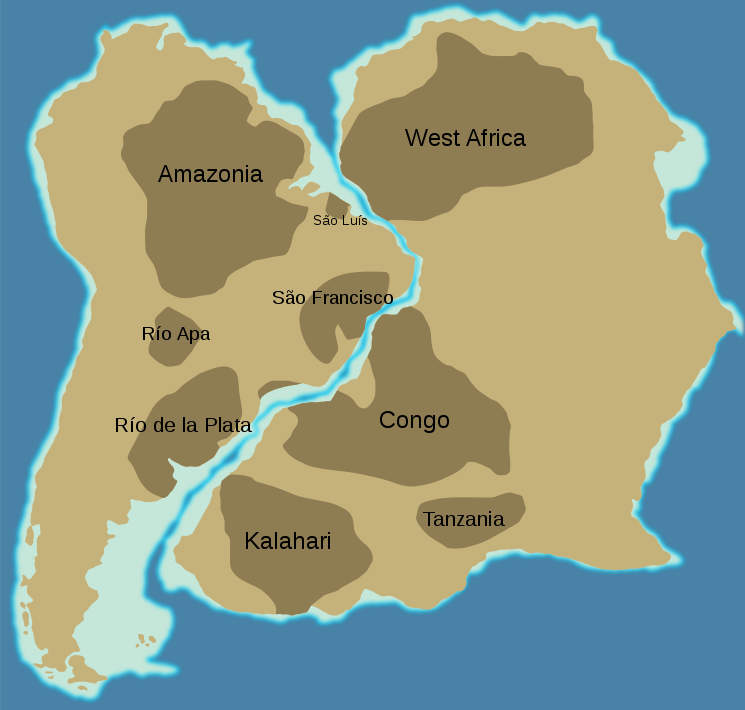
Wegener also referred to the evidence for the Carboniferous and Permian (~300 Ma) Karoo Glaciation in South America, Africa, India, Antarctica, and Australia (Fig. 4.1.4). These areas contain evidence of past glacial deposits, including glacial scars oriented away from the poles, despite the fact that some of these locations are now tropical environments. This indicates that these continents were once closer to the south pole where the glaciers could have formed. Wegener argued that this could only have happened if these continents were once all connected as a single supercontinent. He also cited evidence (based on his own astronomical observations) that showed that the continents were moving with respect to each other, and determined a separation rate between Greenland and Scandinavia of 11 m per year, although he admitted that the measurements were not accurate. In fact they weren’t even close — the separation rate is actually about 2.5 cm per year!
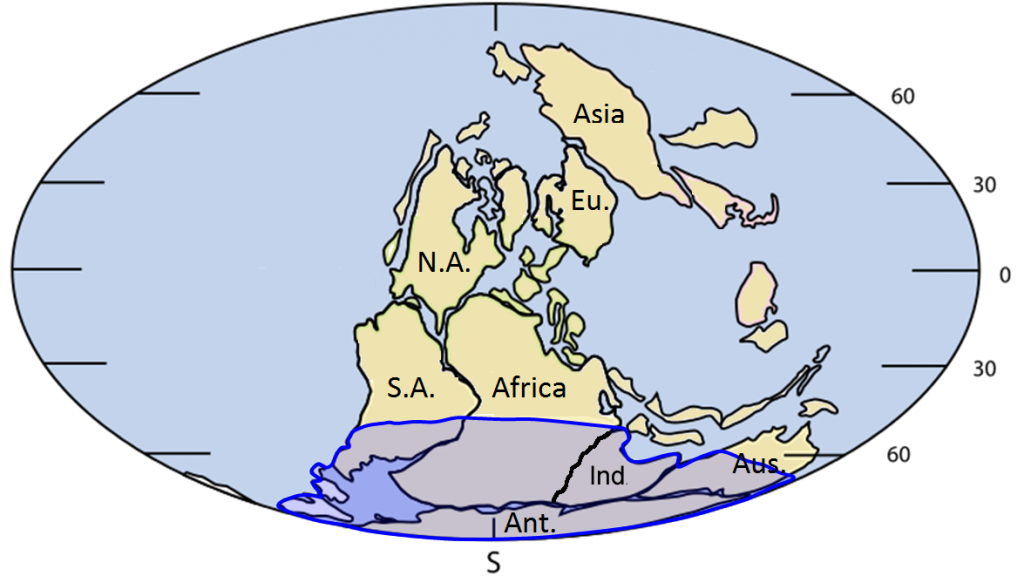
Wegener first published his ideas in 1912 in a short book called Die Entstehung der Kontinente ( The Origin of Continents ), and then in 1915 in Die Entstehung der Kontinente und Ozeane ( The Origin of Continents and Oceans ). He revised this book several times up to 1929, and it was translated into French, English, Spanish, and Russian. However, despite his range of evidence, the continental fits were not perfect and the geological match-ups were not always consistent (while the continental fit left some gaps when using the current coastline, it was demonstrated in the 1960s that using a 500 m depth contour gives a much tighter fit). But the most serious problem of all was that Wegener could not conceive of a good mechanism for moving the continents around. Wegener proposed that the continents were like icebergs floating on heavier crust, but the only forces that he could invoke to propel continents around were poleflucht , the effect of Earth’s rotation pushing objects toward the equator, and the lunar and solar tidal forces, which tend to push objects toward the west. It was quickly shown that these forces were far too weak to move continents, and without any reasonable mechanism to make it work, Wegener’s theory was quickly dismissed by most geologists of the day. Alfred Wegener died in Greenland in 1930 while carrying out studies related to glaciation and climate. At the time of his death, his ideas were tentatively accepted by only a small minority of geologists, and soundly rejected by most. However, within a few decades that was all to change.
Additional links for more information:
- For more about Wegener and the other pioneers of plate tectonics, visit The Geological Society’s Plate Tectonics site: https://www.geolsoc.org.uk/Plate-Tectonics/Chap1-Pioneers-of-Plate-Tectonics
*”Physical Geology” by Steven Earle used under a CC-BY 4.0 international license. Download this book for free at http://open.bccampus.ca
the idea that the continents have moved over the surface of the Earth over geological time (4.1)
the concept that the Earth’s crust and upper mantle (lithosphere) is divided into a number of plates that move independently on the surface and interact with each other at their boundaries (4.1)
a geologic period which spans 47 million years from the end of the Carboniferous Period 298.9 million years ago, to the beginning of the Triassic period 251.902 Mya
the supercontinent that existed between approximately 300 and 180 Ma; it contained all of the modern continents combined into a single land mass (4.1)
a geologic period that spans 60 million years from the end of the Devonian Period 358.9 million years ago, to the beginning of the Permian Period, 298.9 Mya
scratches and grooves carved into bedrock from rocks carried by moving glaciers (4.1)
Introduction to Oceanography Copyright © by Paul Webb is licensed under a Creative Commons Attribution 4.0 International License , except where otherwise noted.
Share This Book
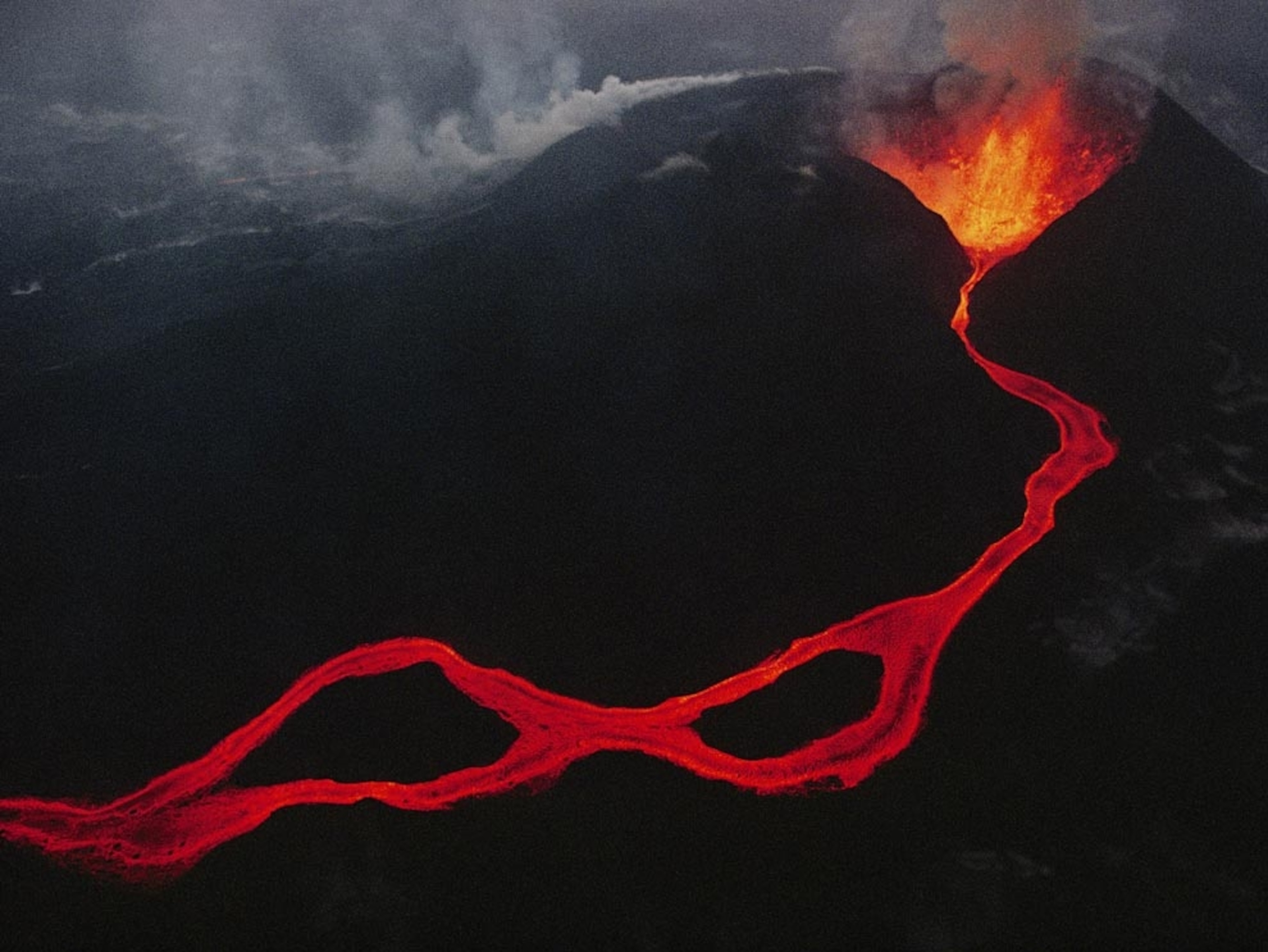
Plate Tectonics
The Earth's plates jostle about in fits and starts that are punctuated with earthquakes and volcanic eruptions.
There are a few handfuls of major plates and dozens of smaller, or minor, plates. Six of the majors are named for the continents embedded within them, such as the North American, African, and Antarctic plates. Though smaller in size, the minors are no less important when it comes to shaping the Earth. The tiny Juan de Fuca plate is largely responsible for the volcanoes that dot the Pacific Northwest of the United States.
The plates make up Earth's outer shell, called the lithosphere . (This includes the crust and uppermost part of the mantle.) Churning currents in the molten rocks below propel them along like a jumble of conveyor belts in disrepair. Most geologic activity stems from the interplay where the plates meet or divide.
The movement of the plates creates three types of tectonic boundaries: convergent, where plates move into one another; divergent, where plates move apart; and transform, where plates move sideways in relation to each other.
They move at a rate of one to two inches (three to five centimeters) per year.
Convergent Boundaries
Where plates serving landmasses collide, the crust crumples and buckles into mountain ranges . India and Asia crashed about 55 million years ago, slowly giving rise to the Himalaya , the highest mountain system on Earth. As the mash-up continues, the mountains get higher. Mount Everest, the highest point on Earth, may be a tiny bit taller tomorrow than it is today.
These convergent boundaries also occur where a plate of ocean dives, in a process called subduction, under a landmass. As the overlying plate lifts up, it also forms mountain ranges. In addition, the diving plate melts and is often spewed out in volcanic eruptions such as those that formed some of the mountains in the Andes of South America.
At ocean-ocean convergences, one plate usually dives beneath the other, forming deep trenches like the Mariana Trench in the North Pacific Ocean, the deepest point on Earth. These types of collisions can also lead to underwater volcanoes that eventually build up into island arcs like Japan.
Divergent Boundaries
At divergent boundaries in the oceans, magma from deep in the Earth's mantle rises toward the surface and pushes apart two or more plates. Mountains and volcanoes rise along the seam. The process renews the ocean floor and widens the giant basins. A single mid-ocean ridge system connects the world's oceans, making the ridge the longest mountain range in the world.
On land, giant troughs such as the Great Rift Valley in Africa form where plates are tugged apart. If the plates there continue to diverge, millions of years from now eastern Africa will split from the continent to form a new landmass. A mid-ocean ridge would then mark the boundary between the plates.
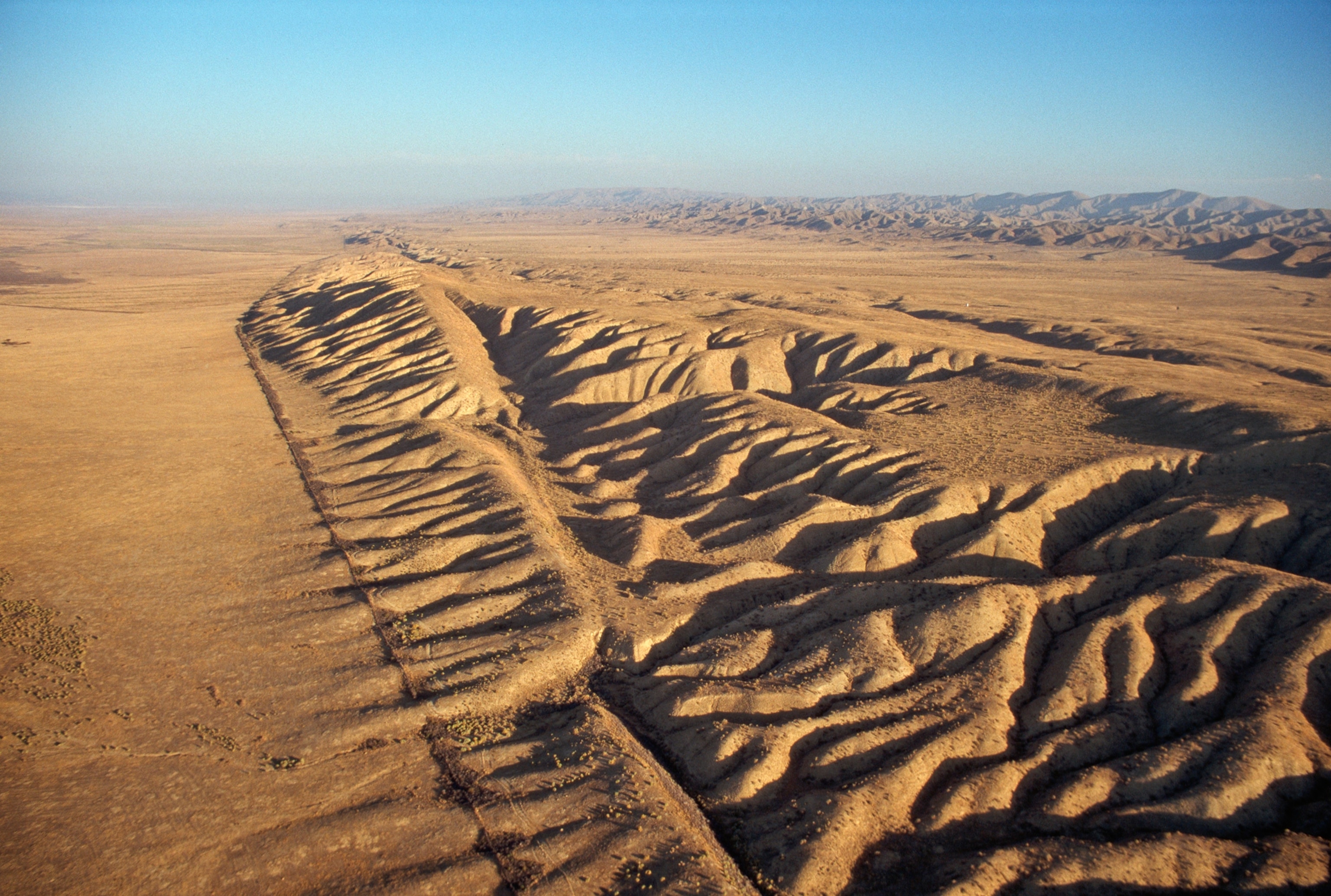
Mountains and a rift can be seen along the San Andreas Fault.
Transform Boundaries
The San Andreas Fault in California is an example of a transform boundary, where two plates grind past each other along what are called strike-slip faults. These boundaries don't produce spectacular features like mountains or oceans, but the halting motion often triggers large earthquakes, such as the 1906 one that devastated San Francisco.
Introducing Nat Geo Kids Book Bundle!
Related topics.
- PLATE TECTONICS
- EARTH SCIENCES
- SUBMARINE VOLCANOES
You May Also Like
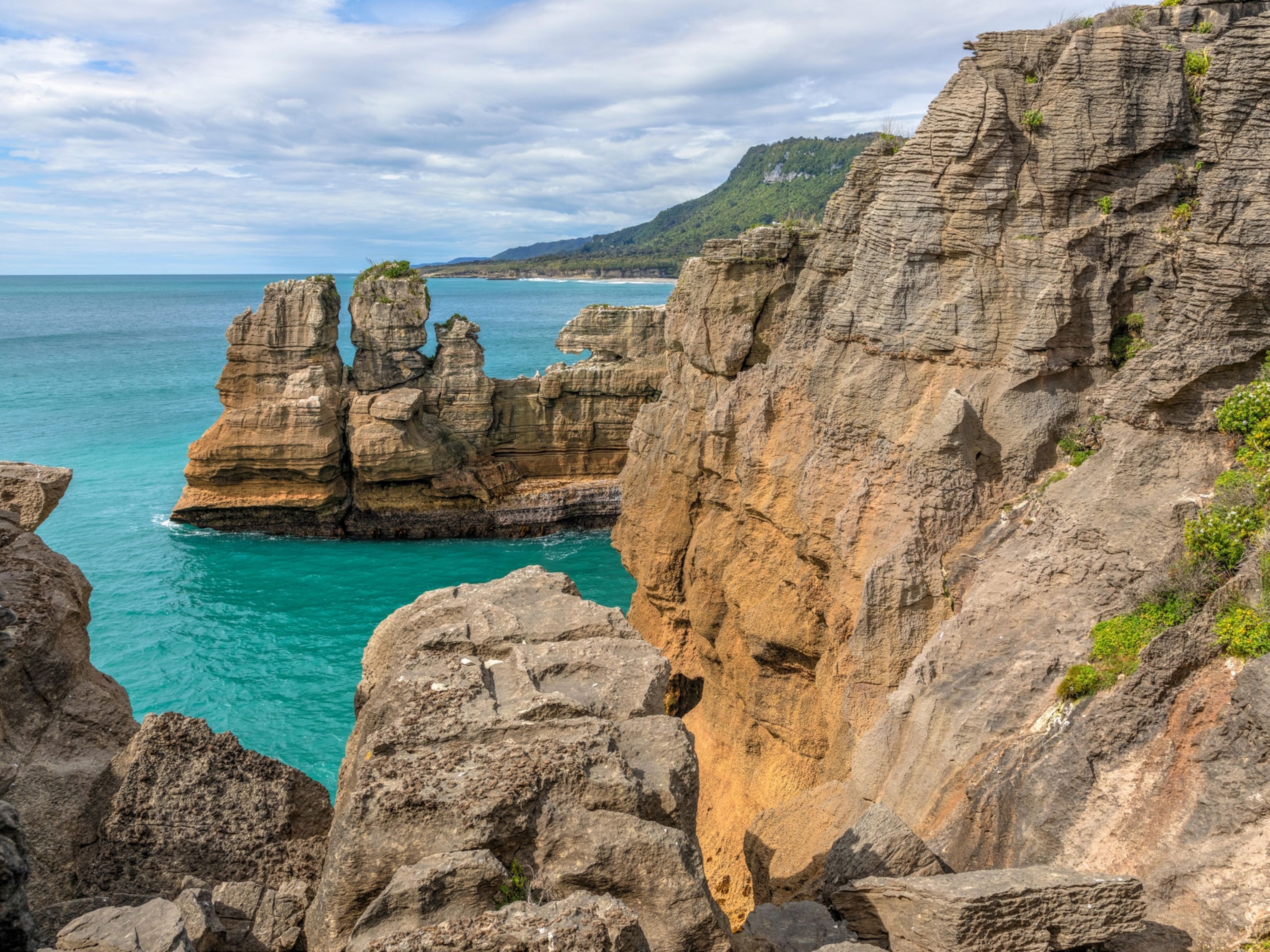

The lost continent of Zealandia has been mapped for the first time
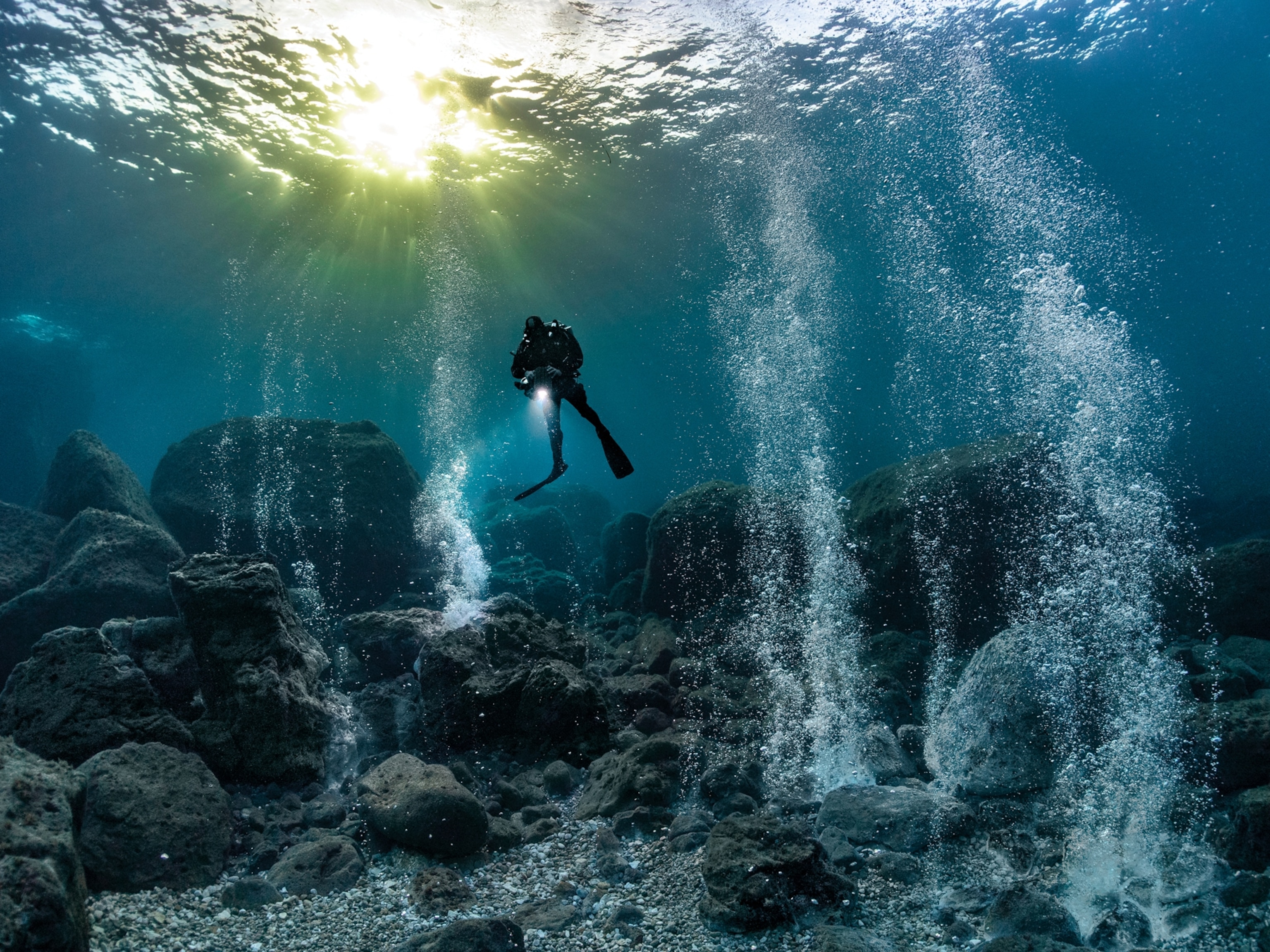
These violent undersea volcanoes harbor a secret: life
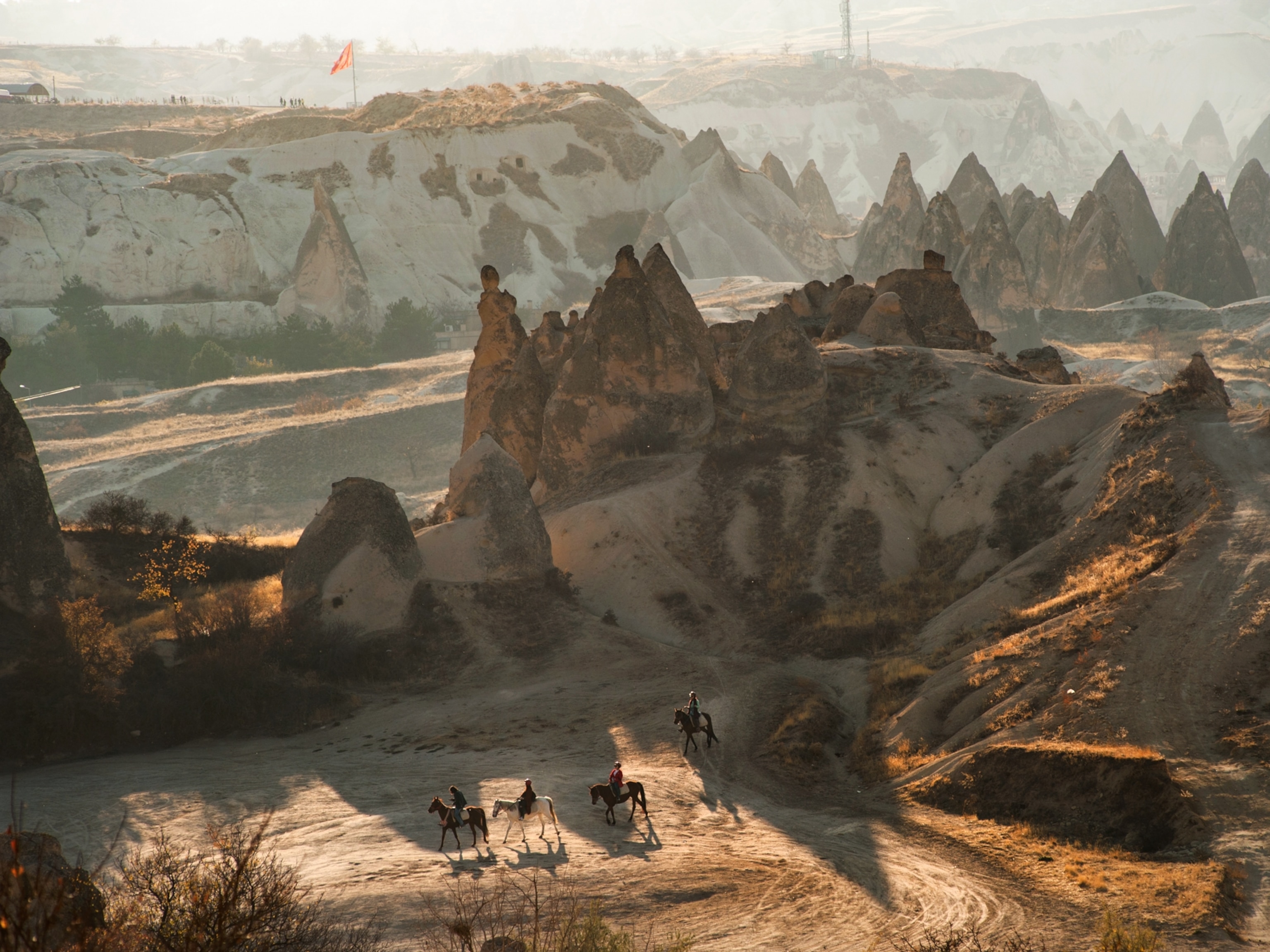
The surprising source of Turkey's volcanoes lies more than 1,000 miles away
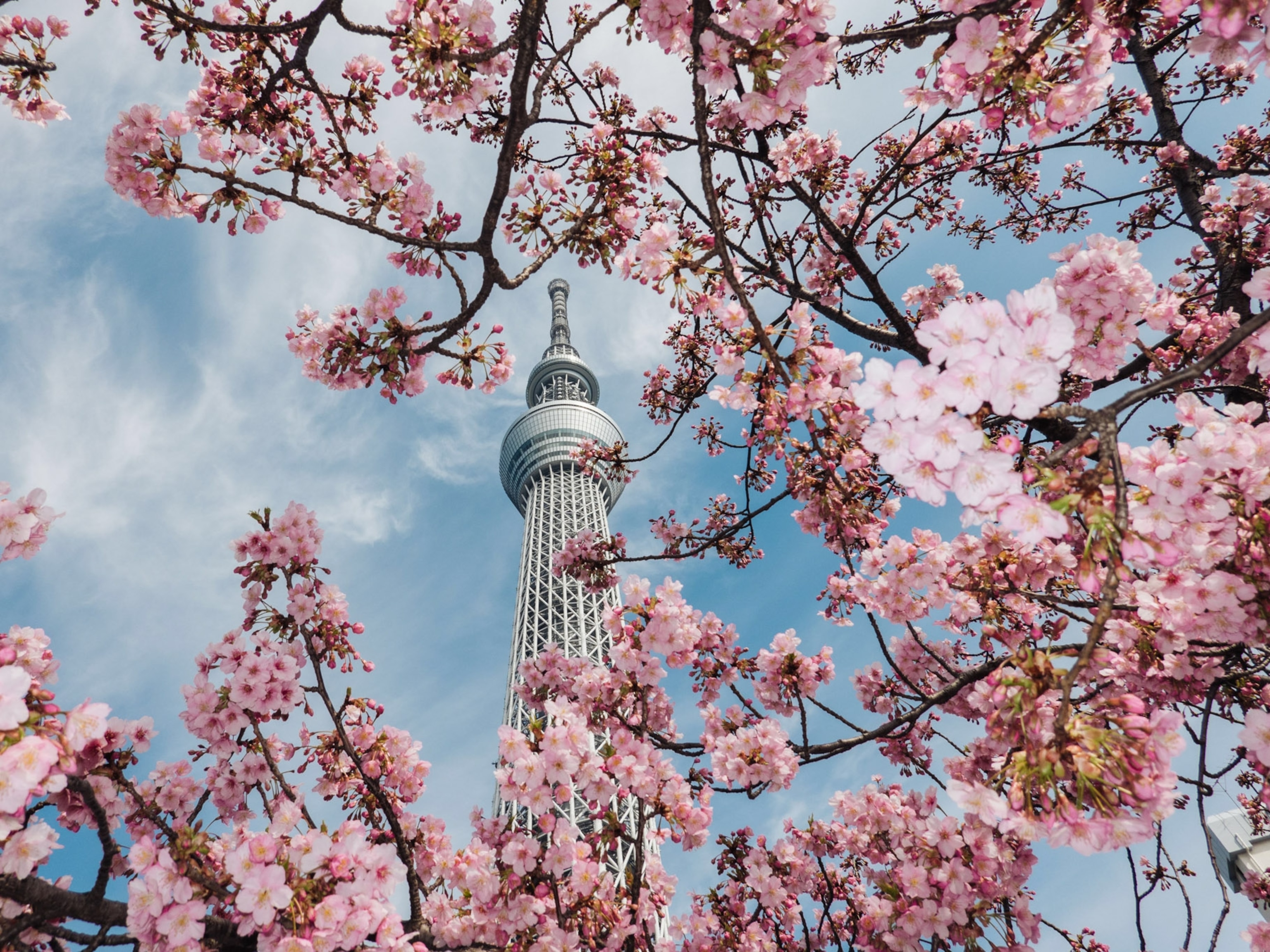
Japan spent decades making itself earthquake resilient. Here's how.
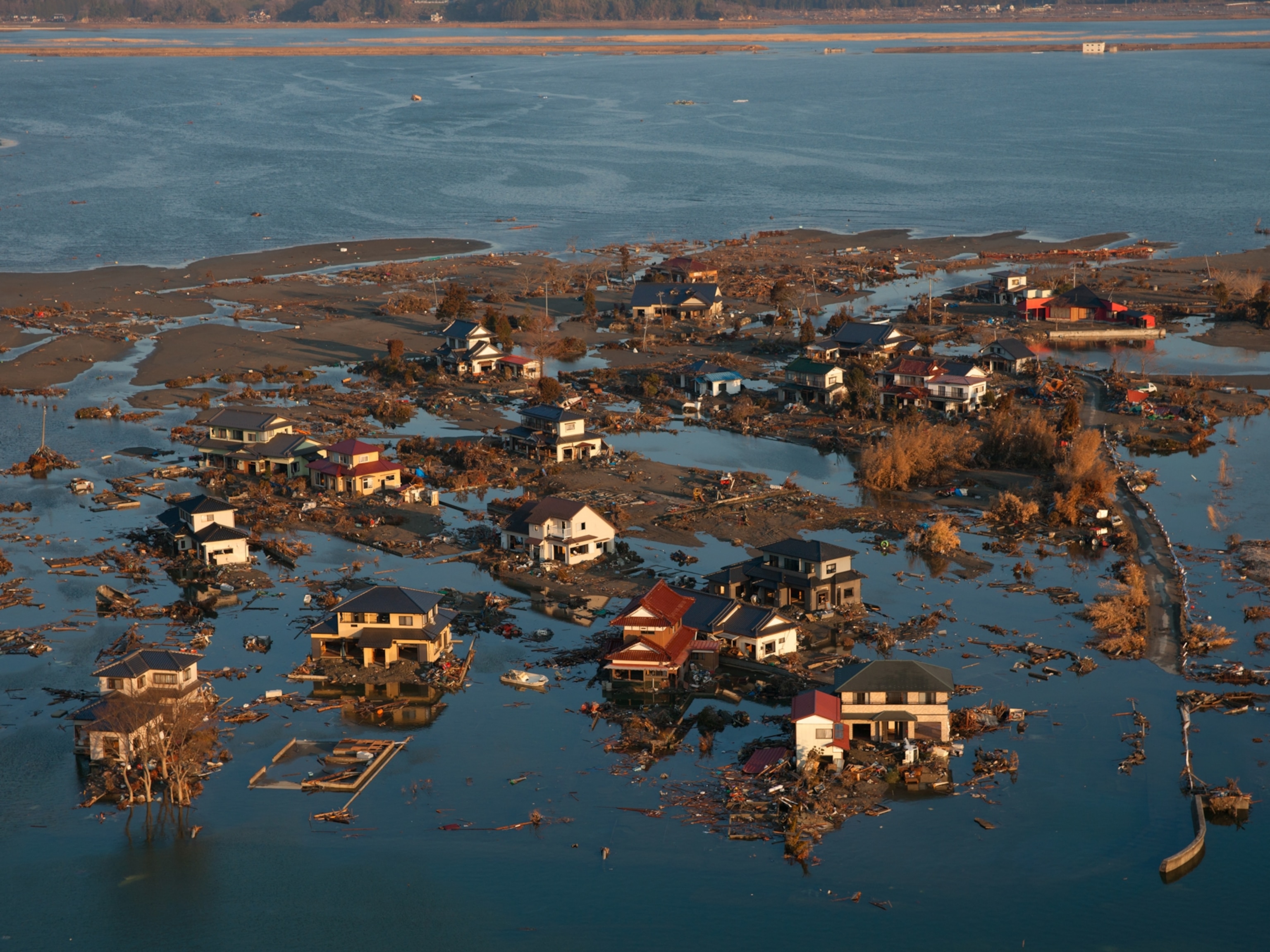
Japan's 2011 megaquake left a scar at the bottom of the sea. Scientists finally explored it.
- Environment
- Paid Content
- Photography
- Perpetual Planet
History & Culture
- History & Culture
- History Magazine
- Mind, Body, Wonder
- Destination Guide
- Terms of Use
- Privacy Policy
- Your US State Privacy Rights
- Children's Online Privacy Policy
- Interest-Based Ads
- About Nielsen Measurement
- Do Not Sell or Share My Personal Information
- Nat Geo Home
- Attend a Live Event
- Book a Trip
- Inspire Your Kids
- Shop Nat Geo
- Visit the D.C. Museum
- Learn About Our Impact
- Support Our Mission
- Advertise With Us
- Customer Service
- Renew Subscription
- Manage Your Subscription
- Work at Nat Geo
- Sign Up for Our Newsletters
- Contribute to Protect the Planet
Copyright © 1996-2015 National Geographic Society Copyright © 2015-2024 National Geographic Partners, LLC. All rights reserved
- The Open University
- Guest user / Sign out
- Study with The Open University
My OpenLearn Profile
Personalise your OpenLearn profile, save your favourite content and get recognition for your learning
About this free course
Become an ou student, download this course, share this free course.

Start this free course now. Just create an account and sign in. Enrol and complete the course for a free statement of participation or digital badge if available.
Plate tectonics is the grand, unifying theory of Earth sciences, combining the concepts of continental drift and sea-floor spreading into one holistic theory that explains many of the major structural features of the Earth's surface. It explains why the oceanic lithosphere is never older than about 180 Ma and why only the continents have preserved the Earth's geological record for the past 4000 Ma. It provides the framework to explain the distribution of earthquakes and volcanoes and a mechanism for the slow drift of the continents across the Earth's surface. The theory has now reached such a level of scientific acceptance that the movement of plates, both relative to one another and to the hot-spot reference frame, are being used to infer movement of the hot-spot reference frame with respect to the Earth's rotational axis.
Plate tectonics is an expression of the convective regime in the underlying mantle, but the link between individual convection cells and plate boundaries is not direct because plate boundaries are not fixed and, like the plates, move relative to one another. Plate movements are driven by gravity, largely by cold, dense lithospheric slabs pulling younger lithosphere towards a destructive boundary. A less-powerful driving force is generated by the potential energy of spreading centres, elevated some 2-3 km above the general level of the abyssal plains.
As ideas concerning plate tectonics have evolved since the 1970s, it has become apparent that while the theory can be applied rigorously to the oceans, the same cannot be said of the continents. Because of the strength and rigidity of oceanic plates, deformation is focused into narrow linear zones along plate margins. By contrast, when continental lithosphere approaches a plate boundary, deformation can extend hundreds of kilometres into the continental interior because continental plates are less strong. Such deformation gives rise to the major mountain belts of the Earth, as exemplified by the Alpine Himalayan Chain.
Plate Tectonics and Natural Disasters
Natural disasters like earthquakes and tsunamis are linked to plate tectonics, the grinding movement of pieces of Earth’s crust.
Earth Science, Geology, Meteorology, Geography, Physical Geography
Damage from the Great Sendai Earthquake
The movement of plate tectonics is not always a slow process. At times it can be fast and violent, causing natural disasters, like the Japan earthquake and tsunami of 2011, also called Great Sendai Earthquake or Great Tohoku Earthquake.
Photograph by Hitoshi Yamada/SIPA
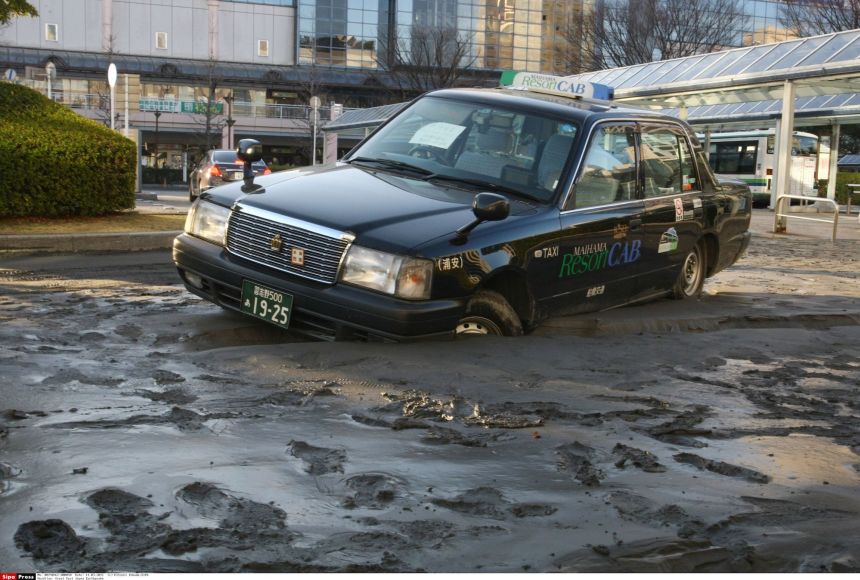
Earth’s surface may look solid—after all, we walk on it and construct buildings on it—but in fact it is a constantly moving puzzle of interlocking pieces. These pieces, known as tectonic plates, are giant sections of Earth’s crust whose edges interact with one another by either colliding or moving apart. The plates of the lithosphere float on top of the malleable asthenosphere in Earth’s interior. The movement of these plates is called plate tectonics, and scientists have studied this field since the 1950s. While the movement of tectonic plates is usually slow—typically just a few centimeters per year—plate tectonics are linked to several kinds of natural disasters , namely earthquakes , volcanoes, and tsunamis . On the afternoon of March 11, 2011, a large earthquake struck off the northeastern coast of Japan. This event, which would prove to be deadly, was caused by a specific type of plate movement: subduction . Subduction occurs when one tectonic plate—the one that is older and denser—sinks or is pulled under another tectonic plate. This process does not proceed smoothly, however—tectonic plates can shift and grind against each other, snagging on each other due to friction. Once plates overcome this friction and move past each other, the energy released leads to earthquakes . Near Japan, the Pacific Plate is subducting under the North American Plate. Although it may seem impossible, parts of Japan actually sit above a portion of the North American Plate. In the 2011 Tohoku Earthquake —so named for the part of northeastern Japan that was struck hardest by the quake—a submerged section of the North American plate jolted upward in the Japan Trench. This undersea valley is located roughly 130 kilometers (81 miles) from the main island of Japan. The magnitude 9.0 earthquake produced by the upward movement of this plate—one of the most powerful quakes in recorded history—hoisted a wall of seawater. That huge upwelling of water created a series of waves—a tsunami — that moved outward in all directions from the earthquake ’s epicenter, both toward and away from Japan. The waves moved at speeds of up to 800 kilometers (500 miles) per hour, roughly the speed of a jet airliner. When those waves rolled up on the eastern shore of Japan, the tallest measured more than 10 meters (33 feet) high. The waves that rushed toward the east eventually struck Hawai'i and then the western coast of the United States, though with much less force. The tsunami that hit Japan was far higher than the seawalls that had been built to protect the Japanese coastline from such inundations. The water rushed inland in a great flood, carrying with it ships, sweeping away cars, and destroying buildings. About 20,000 people were killed. Images captured on the day of the earthquake , as well as the days that followed, revealed a shattered landscape full of debris. The environmental impact of the 2011 earthquake and tsunami has been enormous; researchers studying soil samples have detected pollution from industrial chemicals and pesticides that leaked from the wreckage. That is not surprising given the amount of destruction caused by the disaster: oil refineries in flames, sewer and gas lines broken, and chemical plants damaged. The tsunami also crippled the Fukushima Daiichi Nuclear Power Plant near Sendai, Japan. Ocean waves caused flooding that cut off the plant’s electrical power, making it impossible to cool the plant’s nuclear reactors. As a consequence, three of the plant’s four reactors overheated, causing the uranium fuel rods to liquefy. The melted rods burned through the steel walls meant to contain them, releasing uranium and other radioactive materials into the air and sea. The airborne radioactive particles blanketed houses, crops, and schools. Over 100,000 people were forced to evacuate from their homes. The Japanese government expected to spend the equivalent of more than 200 billion U.S. dollars (and perhaps as much as 600 billion dollars) cleaning up radioactive contamination and dismantling the power station, a task that could take 30 years or more. A lot has been accomplished already, however: 1,500 fuel rods from the Fukushima Daiichi Nuclear Power Plant have been removed and radioactive topsoil and vegetation from the surrounding area have been placed in bags for long-term storage. This earthquake also had far-reaching effects: tsunamis rolled up on distant shorelines in places as far away as Chile, and the intense ground shaking might have even changed the rotation rate of Earth, shortening the length of the day by about 1.8 microseconds. Earthquakes and tsunamis are powerful natural disasters capable of wreaking extreme havoc. For that reason, scientists are interested in being able to predict when and where these events will occur. By installing sensors capable of measuring ground movements, researchers can monitor earthquakes , even tiny ones, worldwide. This data allows scientists to assemble global maps of earthquakes to look for patterns in their locations. Researchers have also placed buoys in the ocean to detect tsunami waves traveling toward land. Detecting a tsunami before it floods a shoreline and issuing an alert can save many lives.
Media Credits
The audio, illustrations, photos, and videos are credited beneath the media asset, except for promotional images, which generally link to another page that contains the media credit. The Rights Holder for media is the person or group credited.
Production Managers
Program specialists, last updated.
October 19, 2023
User Permissions
For information on user permissions, please read our Terms of Service. If you have questions about how to cite anything on our website in your project or classroom presentation, please contact your teacher. They will best know the preferred format. When you reach out to them, you will need the page title, URL, and the date you accessed the resource.
If a media asset is downloadable, a download button appears in the corner of the media viewer. If no button appears, you cannot download or save the media.
Text on this page is printable and can be used according to our Terms of Service .
Interactives
Any interactives on this page can only be played while you are visiting our website. You cannot download interactives.
Related Resources

- August 2024
- September 2024
- Metamorphic
- Sedimentary
Driving Forces of Plate Motion
Driving forces related to mantle dynamics, driving forces related to gravity, driving forces related to earth rotation, popular this week.

What is Green Obsidian
%20(1).webp)
Types of Drainage Patterns
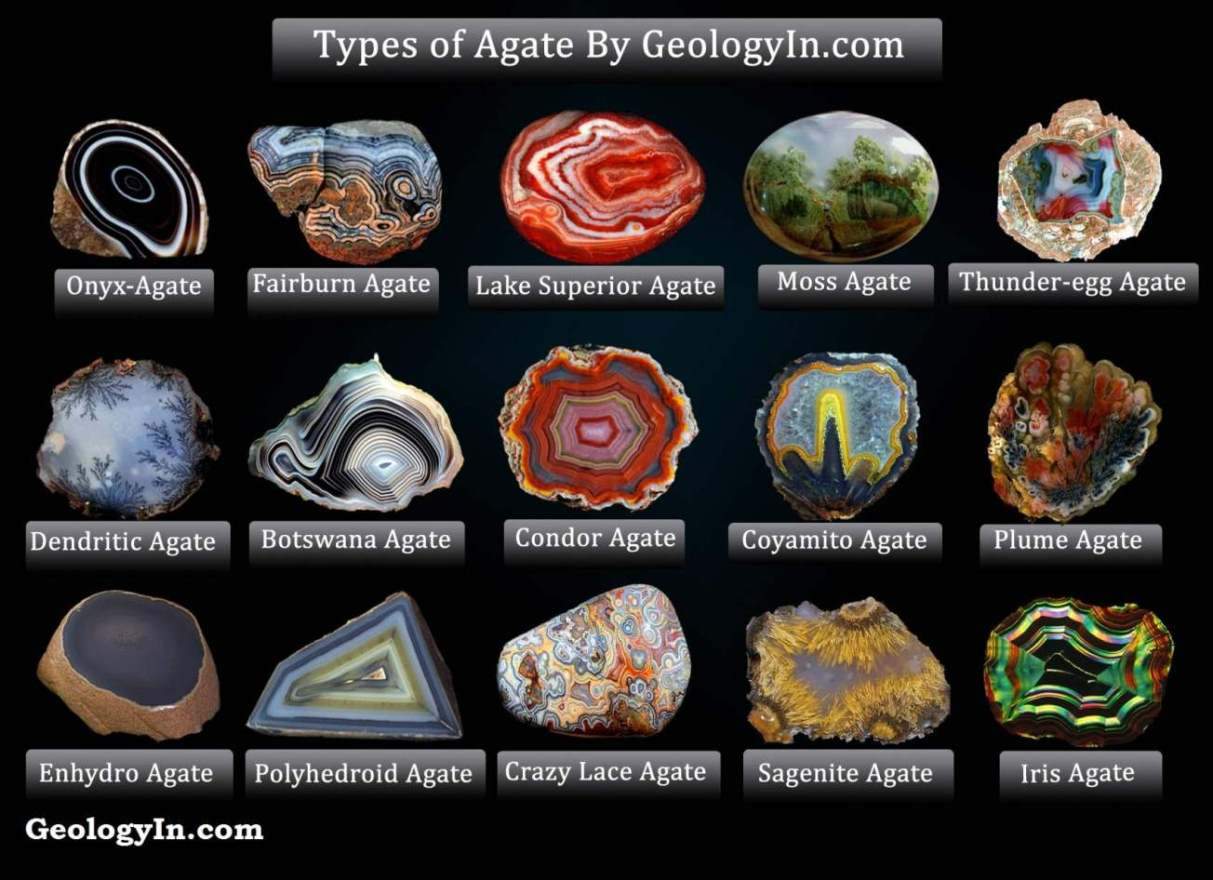
Types of Agate With Photos
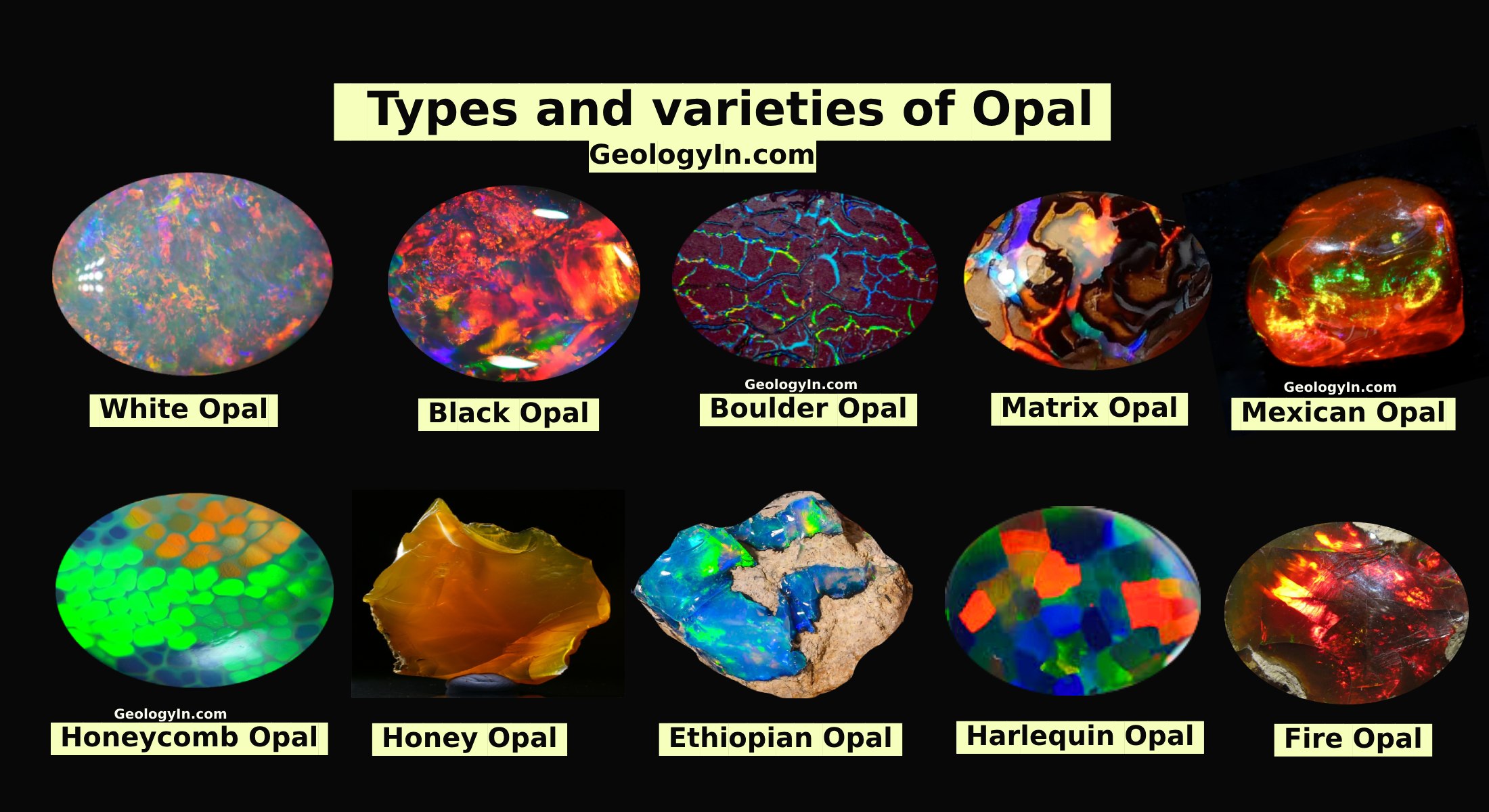
Types of Opal With Photos

Carnelian: Fiery Gemstone

Mystic Topaz: Enhanced Brilliance
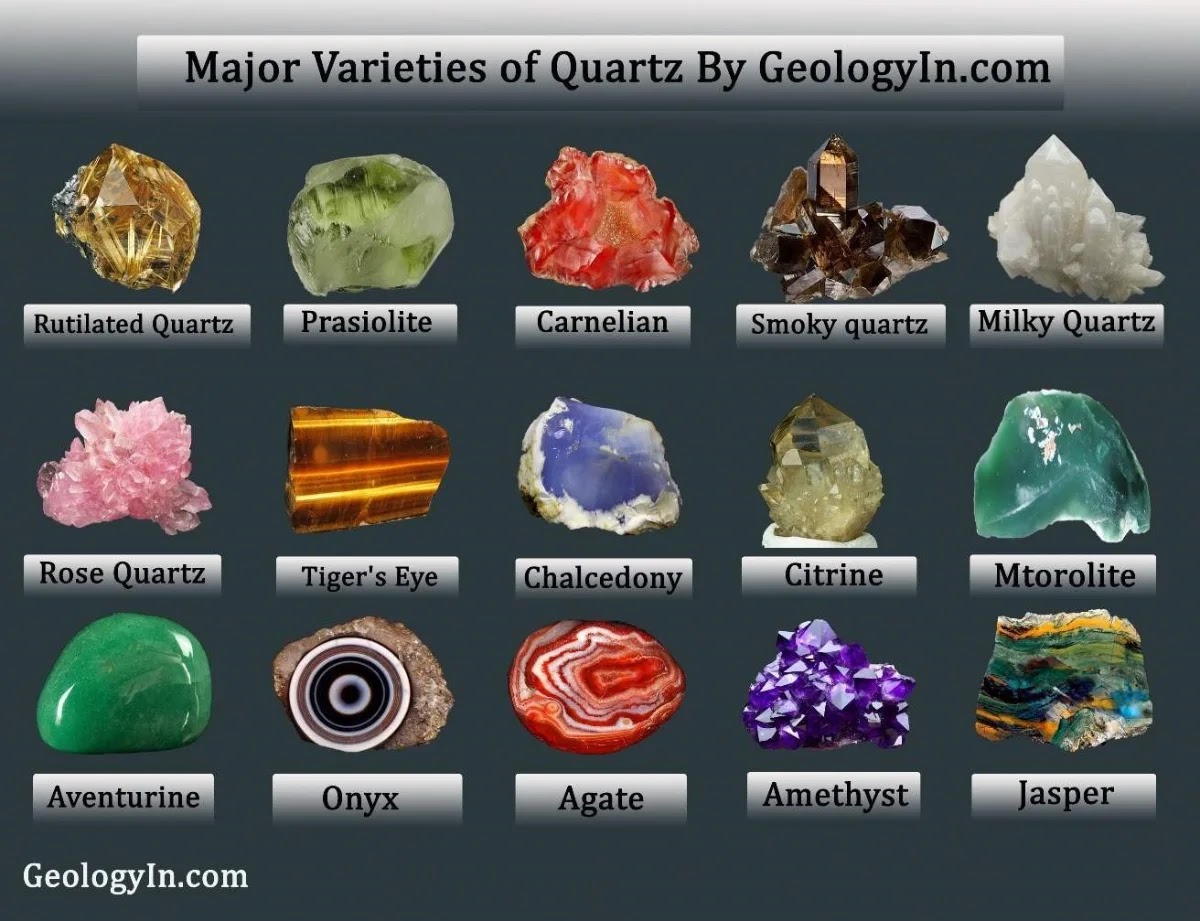
Quartz: Types and Varieties of Quartz (Photos)

The World’s Deepest Cave Known As ‘Everest Of The Caves’
- Gem Hunting In the US
- New York Gem Mining
- Montana Gem Mining
- North Carolina Gem Mining
- California Gem Mining
- Arkansas Gem Mining
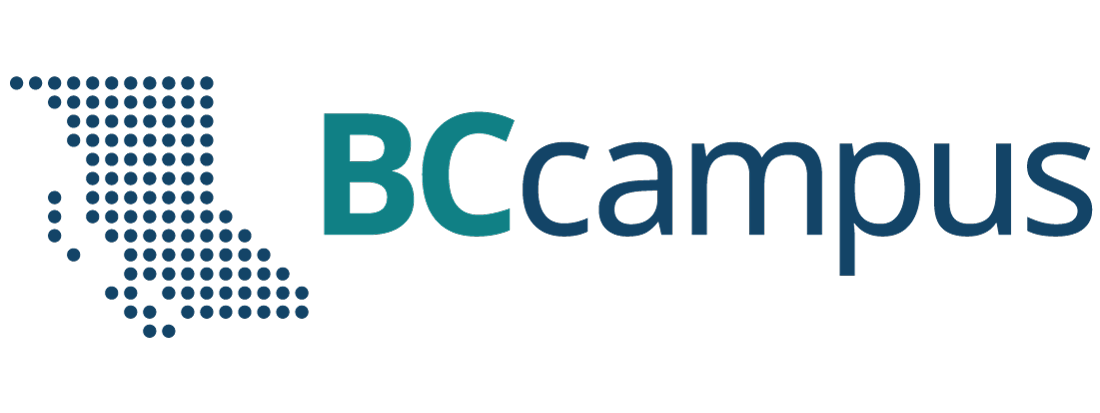
Want to create or adapt books like this? Learn more about how Pressbooks supports open publishing practices.
Chapter 10 Plate Tectonics
10.4 Plate, Plate Motions, and Plate Boundary Processes
Continental drift and sea-floor spreading became widely accepted around 1965 as more and more geologists started thinking in these terms. By the end of 1967 the Earth’s surface had been mapped into a series of plates (Figure 10.4.1). The major plates are Eurasia, Pacific, India, Australia, North America, South America, Africa, and Antarctic. There are also numerous small plates (e.g., Juan de Fuca, Cocos, Nazca, Scotia, Philippine, Caribbean), and many very small plates or sub-plates. For example the Juan de Fuca Plate is actually three separate plates (Gorda, Juan de Fuca, and Explorer) that all move in the same general direction but at slightly different rates.
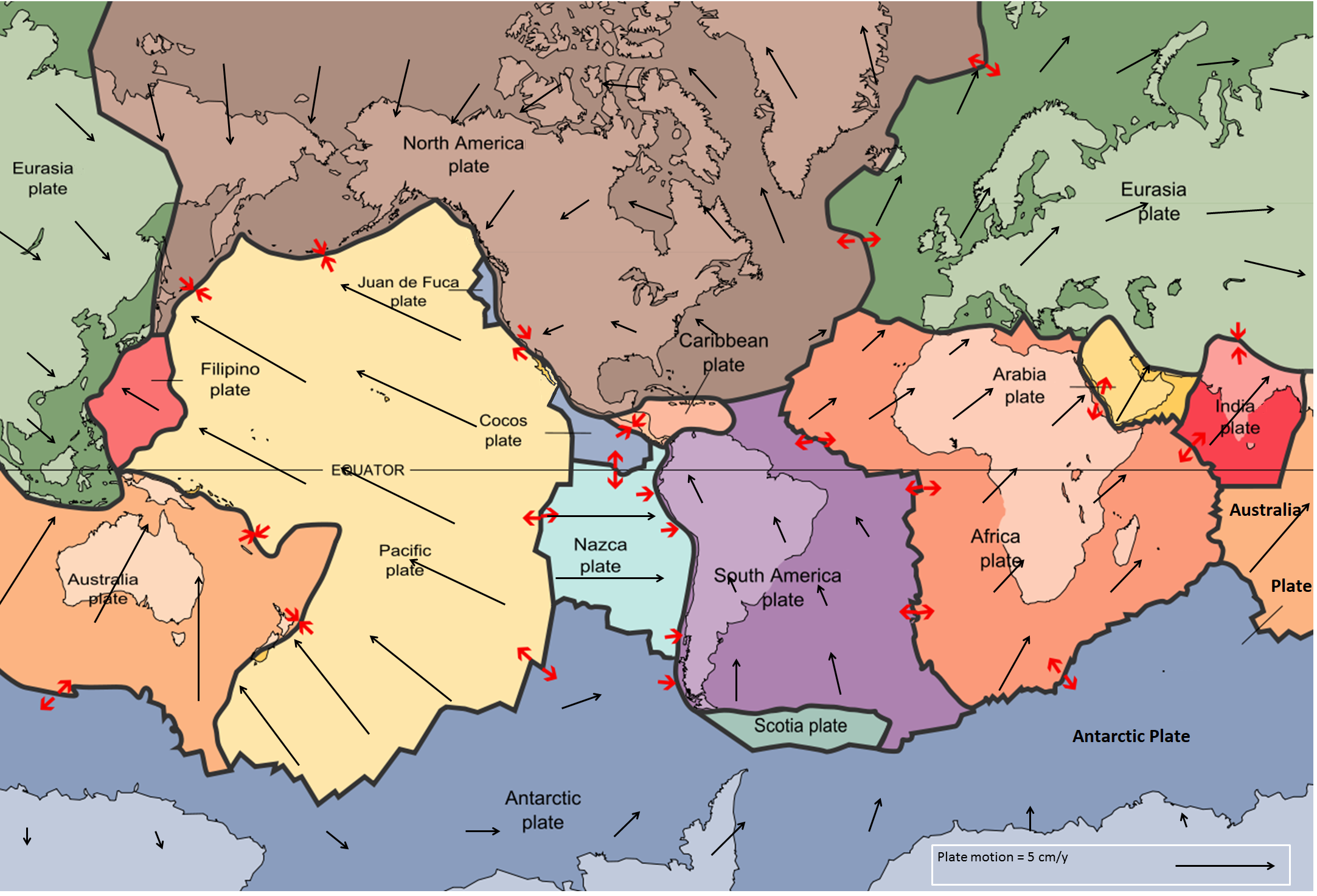
Rates of motions of the major plates range from less than 1 cm/y to over 10 cm/y. The Pacific Plate is the fastest, followed by the Australian and Nazca Plates. The North American Plate is one of the slowest, averaging around 1 cm/y in the south up to almost 4 cm/y in the north.
Plates move as rigid bodies, so it may seem surprising that the North American Plate can be moving at different rates in different places. The explanation is that plates move in a rotational manner. The North American Plate, for example, rotates counter-clockwise; the Eurasian Plate rotates clockwise.
Boundaries between the plates are of three types: divergent (i.e., moving apart) , convergent (i.e., moving together), and transform (moving side by side). Before we talk about processes at plate boundaries, it’s important to point out that there are never gaps between plates. The plates are made up of crust and the lithospheric part of the mantle (Figure 10.4.2), and even though they are moving all the time, and in different directions, there is never a significant amount of space between them. Plates are thought to move along the lithosphere-asthenosphere boundary, as the asthenosphere is the zone of partial melting. It is assumed that the relative lack of strength of the partial melting zone facilitates the sliding of the lithospheric plates.
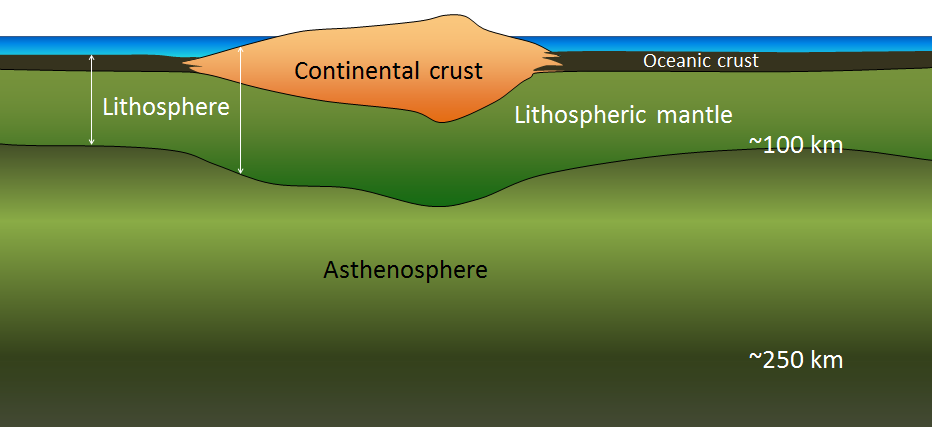
At spreading centres, the lithospheric mantle may be very thin because the upward convective motion of hot mantle material generates temperatures that are too high for the existence of a significant thickness of rigid lithosphere (Figure 10.3.8). The fact that the plates include both crustal material and lithospheric mantle material makes it possible for a single plate to be made up of both oceanic and continental crust. For example, the North American Plate includes most of North America, plus half of the northern Atlantic Ocean. Similarly the South American Plate extends across the western part of the southern Atlantic Ocean, while the European and African plates each include part of the eastern Atlantic Ocean. The Pacific Plate is almost entirely oceanic, but it does include the part of California west of the San Andreas Fault.
Divergent Boundaries
Divergent boundaries are spreading boundaries, where new oceanic crust is created from magma derived from partial melting of the mantle caused by decompression as hot mantle rock from depth is moved toward the surface (Figure 10.4.3). The triangular zone of partial melting near the ridge crest is approximately 60 km thick and the proportion of magma is about 10% of the rock volume, thus producing crust that is about 6 km thick. Most divergent boundaries are located at the oceanic ridges (although some are on land), and the crustal material created at a spreading boundary is always oceanic in character; in other words, it is mafic igneous rock (e.g., basalt or gabbro, rich in ferromagnesian minerals). Spreading rates vary considerably, from 2 cm/y to 6 cm/y in the Atlantic, to between 12 cm/y and 20 cm/y in the Pacific. (Note that spreading rates are typically double the velocities of the two plates moving away from a ridge.)
Some of the processes taking place in this setting include:
- Magma from the mantle pushing up to fill the voids left by divergence of the two plates
- Pillow lavas forming where magma is pushed out into seawater (Figure 10.4.4)
- Vertical sheeted dykes intruding into cracks resulting from the spreading
- Magma cooling more slowly in the lower part of the new crust and forming gabbro bodies
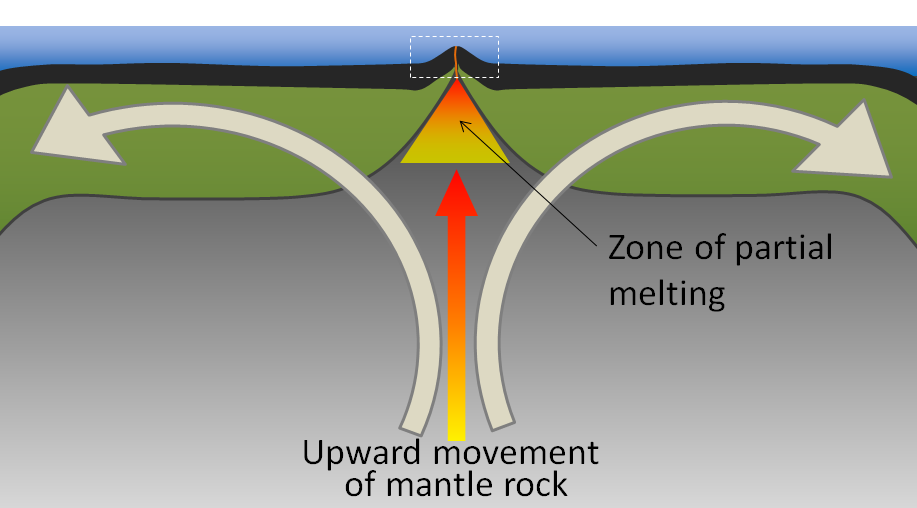
Spreading is hypothesized to start within a continental area with up-warping or doming related to an underlying mantle plume or series of mantle plumes. The buoyancy of the mantle plume material creates a dome within the crust, causing it to fracture in a radial pattern, with three arms spaced at approximately 120° (Figure 10.4.5). When a series of mantle plumes exists beneath a large continent, the resulting rifts may align and lead to the formation of a rift valley (such as the present-day Great Rift Valley in eastern Africa). It is suggested that this type of valley eventually develops into a linear sea (such as the present-day Red Sea), and finally into an ocean (such as the Atlantic). It is likely that as many as 20 mantle plumes, many of which still exist, were responsible for the initiation of the rifting of Pangea along what is now the mid-Atlantic ridge (see Figure 10.3.10).
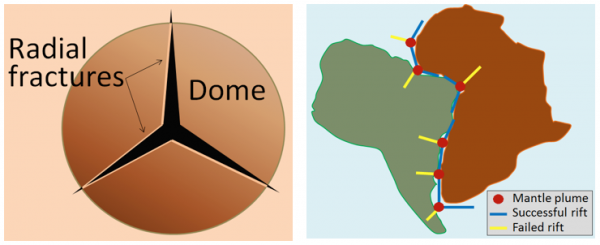
Convergent Boundaries
Convergent boundaries, where two plates are moving toward each other, are of three types, depending on whether oceanic or continental crust is present on either side of the boundary. The types are ocean-ocean, ocean-continent, and continent-continent.
At an ocean-ocean convergent boundary, one of the plates (oceanic crust and lithospheric mantle) is pushed, or subducted , under the other. Often it is the older and colder plate that is denser and subducts beneath the younger and hotter plate. There is commonly an ocean trench along the boundary. The subducted lithosphere descends into the hot mantle at a relatively shallow angle close to the subduction zone, but at steeper angles farther down (up to about 45°). As discussed in the context of subduction-related volcanism in Chapter 4, the significant volume of water within the subducting material is released as the subducting crust is heated. Most of this water is present within the sheet silicate mineral serpentine which is derived from alteration of pyroxene and olivine near the spreading ridge shortly after the rock’s formation. It is released when the oceanic crust is heats and then rises and mixes with the overlying mantle. The addition of water to the hot mantle lowers the rocks’s melting point and leads to the formation of magma (flux melting) (Figure 10.4.6). The magma, which is lighter than the surrounding mantle material, rises through the mantle and the overlying oceanic crust to the ocean floor where it creates a chain of volcanic islands known as an island arc. A mature island arc develops into a chain of relatively large islands (such as Japan or Indonesia) as more and more volcanic material is extruded and sedimentary rocks accumulate around the islands.
As described above in the context of Benioff zones (Figure 10.3.6), earthquakes take place close to the boundary between the subducting crust and the overriding crust. The largest earthquakes occur near the surface where the subducting plate is still cold and strong.
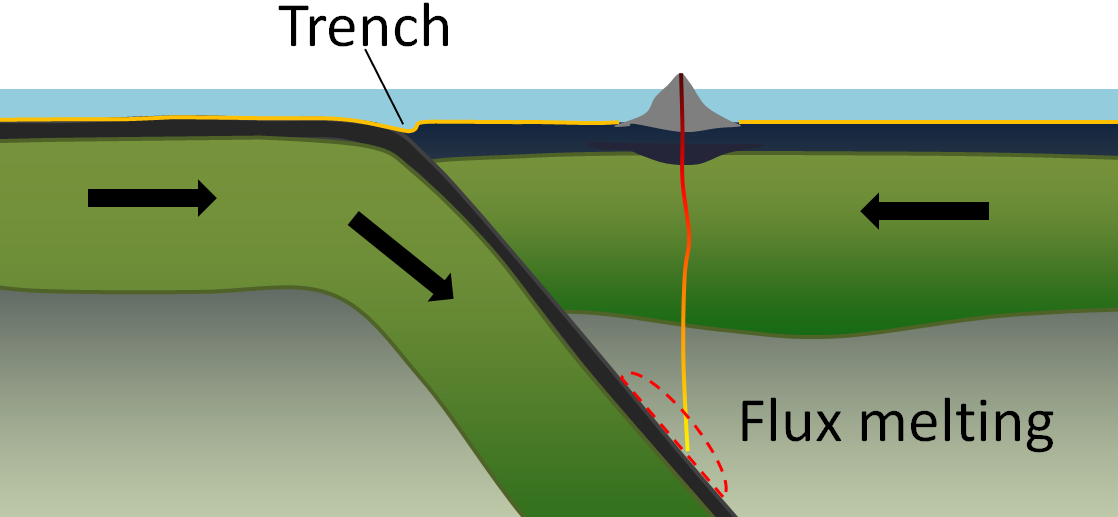
Examples of ocean-ocean convergent zones are subduction of the Pacific Plate beneath the North America Plate south of Alaska (Aleutian Islands) and beneath the Philippine Plate west of the Philippines, subduction of the India Plate beneath the Eurasian Plate south of Indonesia, and subduction of the Atlantic Plate beneath the Caribbean Plate (see Figure 10.4.1).
At an ocean-continent convergent boundary, the oceanic plate is pushed under the continental plate in the same manner as at an ocean-ocean boundary. Sediment that has accumulated on the continental slope is thrust up into an accretionary wedge, and compression leads to thrusting within the continental plate (Figure 10.4.7). The mafic magma produced adjacent to the subduction zone rises to the base of the continental crust and leads to partial melting of the crustal rock. The resulting magma ascends through the crust, producing a mountain chain with many volcanoes.
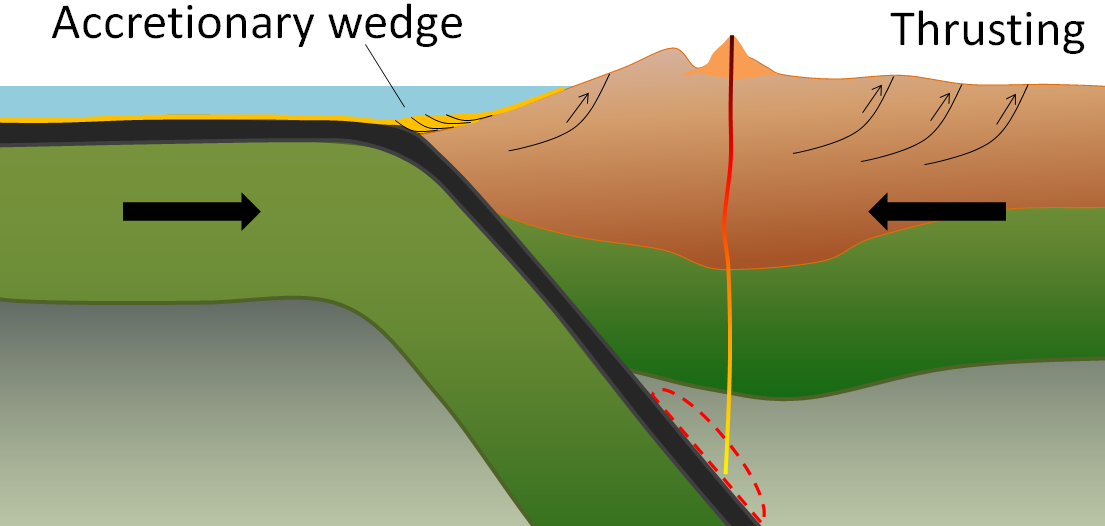
Examples of ocean-continent convergent boundaries are subduction of the Nazca Plate under South America (which has created the Andes Range) and subduction of the Juan de Fuca Plate under North America (creating the mountains Garibaldi, Baker, St. Helens, Rainier, Hood, and Shasta, collectively known as the Cascade Range).
A continent-continent collision occurs when a continent or large island that has been moved along with subducting oceanic crust collides with another continent (Figure 10.4.8). The colliding continental material will not be subducted because it is too light (i.e., because it is composed largely of light continental rocks [SIAL]), but the root of the oceanic plate will eventually break off and sink into the mantle. There is tremendous deformation of the pre-existing continental rocks, and creation of mountains from that rock, from any sediments that had accumulated along the shores (i.e., within geosynclines) of both continental masses, and commonly also from some ocean crust and upper mantle material.
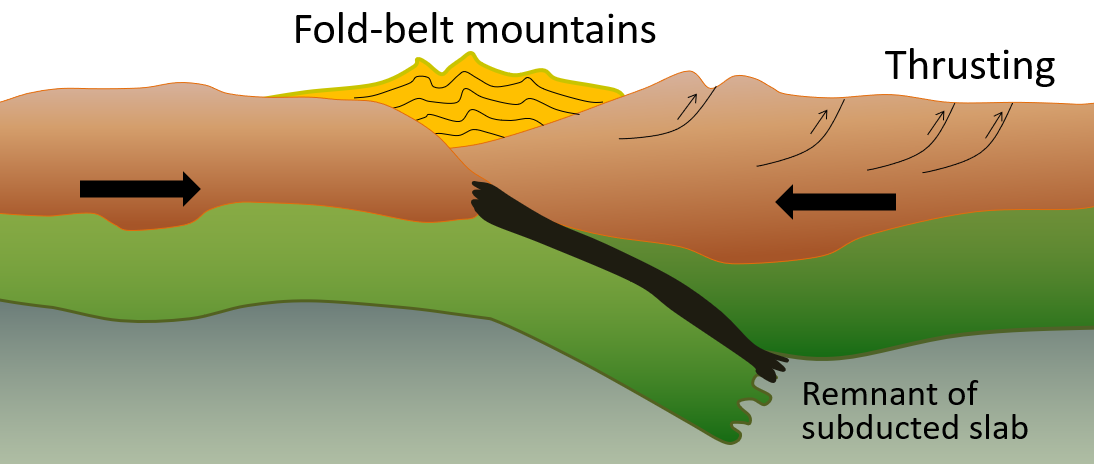
Examples of continent-continent convergent boundaries are the collision of the India Plate with the Eurasian Plate, creating the Himalaya Mountains, and the collision of the African Plate with the Eurasian Plate, creating the series of ranges extending from the Alps in Europe to the Zagros Mountains in Iran. The Rocky Mountains in B.C. and Alberta are also a result of continent-continent collisions.
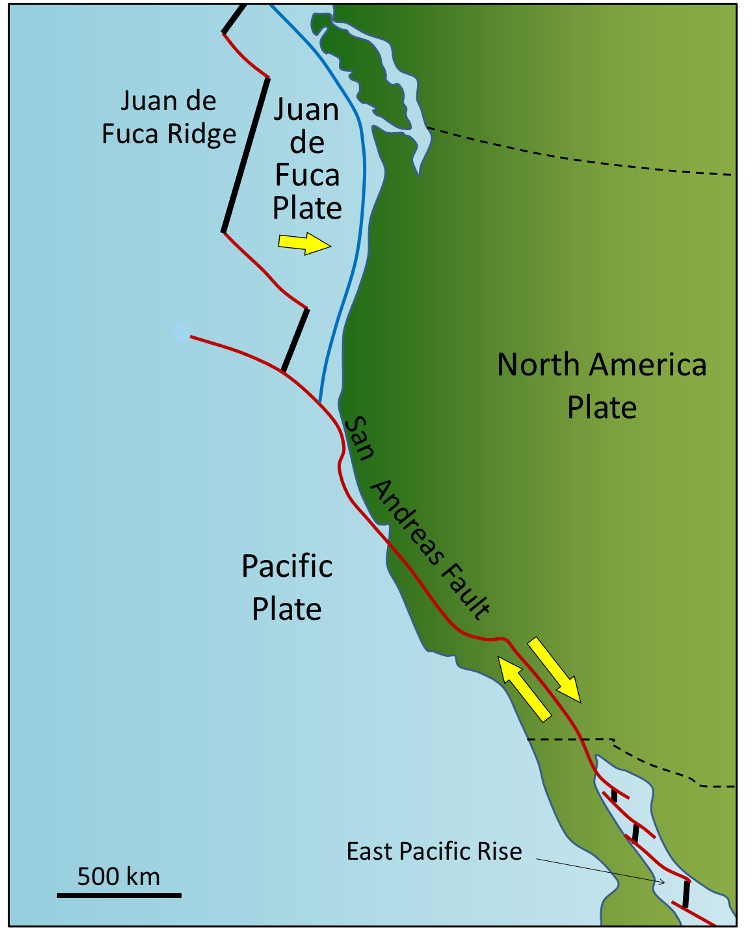
Transform boundaries exist where one plate slides past another without production or destruction of crustal material. As explained above, most transform faults connect segments of mid-ocean ridges and are thus ocean-ocean plate boundaries (Figure 10.3.11). Some transform faults connect continental parts of plates. An example is the San Andreas Fault, which extends from the southern end of the Juan de Fuca Ridge to the northern end of the East Pacific Rise (ridge) in the Gulf of California (Figures 10.28 and 10.29). The part of California west of the San Andreas Fault and all of Baja California are on the Pacific Plate. Transform faults do not just connect divergent boundaries. For example, the Queen Charlotte Fault connects the north end of the Juan de Fuca Ridge, starting at the north end of Vancouver Island, to the Aleutian subduction zone.
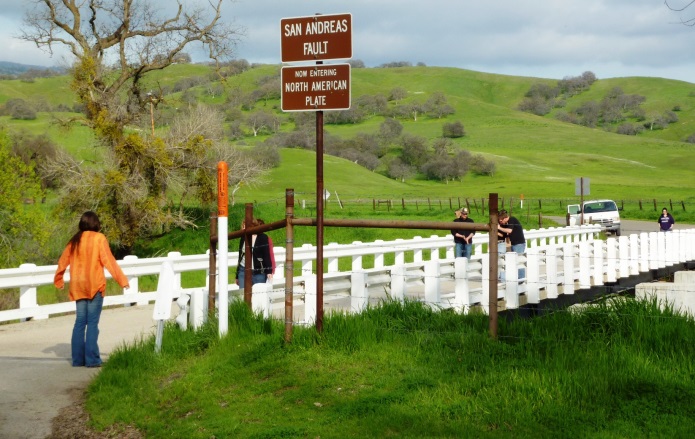
Exercise 10.4 A different type of transform fault
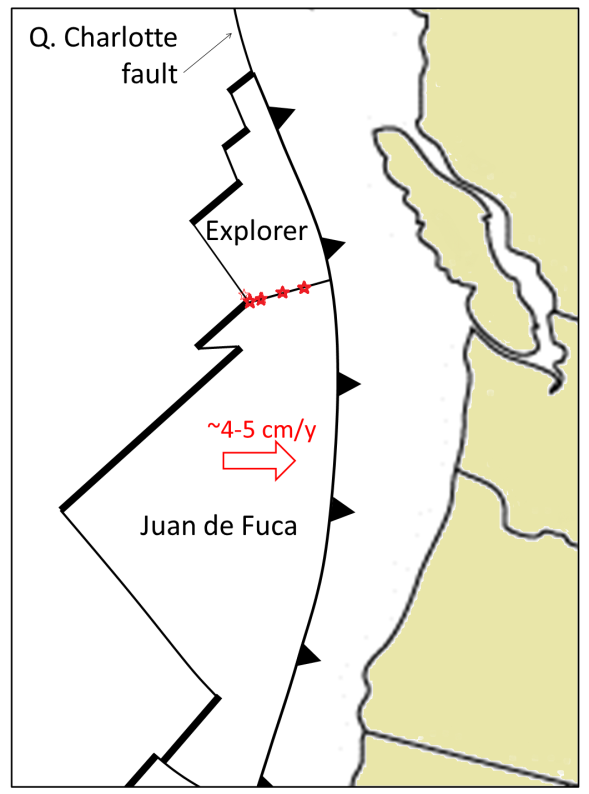
This map shows the Juan de Fuca (JDF) and Explorer Plates off the coast of Vancouver Island. We know that the JDF Plate is moving toward the North American Plate at around 4 centimetres per year to 5 centimetres per year. We think that the Explorer Plate is also moving east, but we don’t know the rate, and there is evidence that it is slower than the JDF Plate.
The boundary between the two plates is the Nootka Fault, which is the location of frequent small-to-medium earthquakes (roughly up to magnitude 5), as depicted by the red stars. Explain why the Nootka Fault is a transform fault, and show the relative sense of motion along the fault with two small arrows.
See Appendix 3 for Exercise 10.4 answers .
As originally described by Wegener in 1915, the present continents were once all part of a supercontinent, which he termed Pangea (meaning all land ). More recent studies of continental matchups and the magnetic ages of ocean-floor rocks have enabled us to reconstruct the history of the break-up of Pangea.
Pangea began to rift apart along a line between Africa and Asia and between North America and South America at around 200 Ma. During the same period, the Atlantic Ocean began to open up between northern Africa and North America, and India broke away from Antarctica. Between 200 and 150 Ma, rifting started between South America and Africa and between North America and Europe, and India moved north toward Asia. By 80 Ma, Africa had separated from South America, most of Europe had separated from North America, and India had separated from Antarctica. By 50 Ma, Australia had separated from Antarctic, and shortly after that, India collided with Asia. To see the timing of these processes for yourself, go to time lapse of Continental Movements .
Within the past few million years, rifting has taken place in the Gulf of Aden and the Red Sea, and also within the Gulf of California. Incipient rifting has begun along the Great Rift Valley of eastern Africa, extending from Ethiopia and Djibouti on the Gulf of Aden (Red Sea) all the way south to Malawi.
Over the next 50 million years, it is likely that there will be full development of the east African rift and creation of new ocean floor. Eventually Africa will split apart. There will also be continued northerly movement of Australia and Indonesia. The western part of California (including Los Angeles and part of San Francisco) will split away from the rest of North America, and eventually sail right by the west coast of Vancouver Island, en route to Alaska. Because the oceanic crust formed by spreading on the mid-Atlantic ridge is not currently being subducted (except in the Caribbean), the Atlantic Ocean is slowly getting bigger, and the Pacific Ocean is getting smaller. If this continues without changing for another couple hundred million years, we will be back to where we started, with one supercontinent.
Pangea, which existed from about 350 to 200 Ma, was not the first supercontinent. It was preceded by Pannotia (600 to 540 Ma), by Rodinia (1,100 to 750 Ma), and by others before that.
In 1966, Tuzo Wilson proposed that there has been a continuous series of cycles of continental rifting and collision; that is, break-up of supercontinents, drifting, collision, and formation of other supercontinents. At present, North and South America, Europe, and Africa are moving with their respective portions of the Atlantic Ocean. The eastern margins of North and South America and the western margins of Europe and Africa are called passive margins because there is no subduction taking place along them.
This situation may not continue for too much longer, however. As the Atlantic Ocean floor gets weighed down around its margins by great thickness of continental sediments (i.e., geosynclines), it will be pushed farther and farther into the mantle, and eventually the oceanic lithosphere may break away from the continental lithosphere (Figure 10.4.12). A subduction zone will develop, and the oceanic plate will begin to descend under the continent. Once this happens, the continents will no longer continue to move apart because the spreading at the mid-Atlantic ridge will be taken up by subduction. If spreading along the mid-Atlantic ridge continues to be slower than spreading within the Pacific Ocean, the Atlantic Ocean will start to close up, and eventually (in a 100 million years or more) North and South America will collide with Europe and Africa.
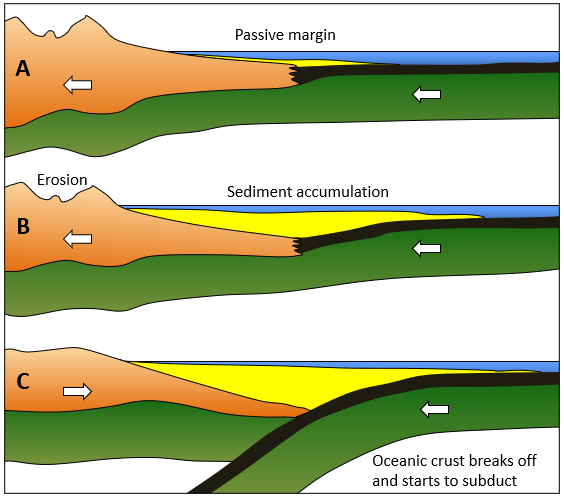
There is strong evidence around the margins of the Atlantic Ocean that this process has taken place before. The roots of ancient mountain belts, which are present along the eastern margin of North America, the western margin of Europe, and the northwestern margin of Africa, show that these land masses once collided with each other to form a mountain chain, possibly as big as the Himalayas. The apparent line of collision runs between Norway and Sweden, between Scotland and England, through Ireland, through Newfoundland, and the Maritimes, through the northeastern and eastern states, and across the northern end of Florida. When rifting of Pangea started at approximately 200 Ma, the fissuring was along a different line from the line of the earlier collision. This is why some of the mountain chains formed during the earlier collision can be traced from Europe to North America and from Europe to Africa.
That the Atlantic Ocean rift may have occurred in approximately the same place during two separate events several hundred million years apart is probably no coincidence. The series of hot spots that has been identified in the Atlantic Ocean may also have existed for several hundred million years, and thus may have contributed to rifting in roughly the same place on at least two separate occasions (Figure 10.3.13).
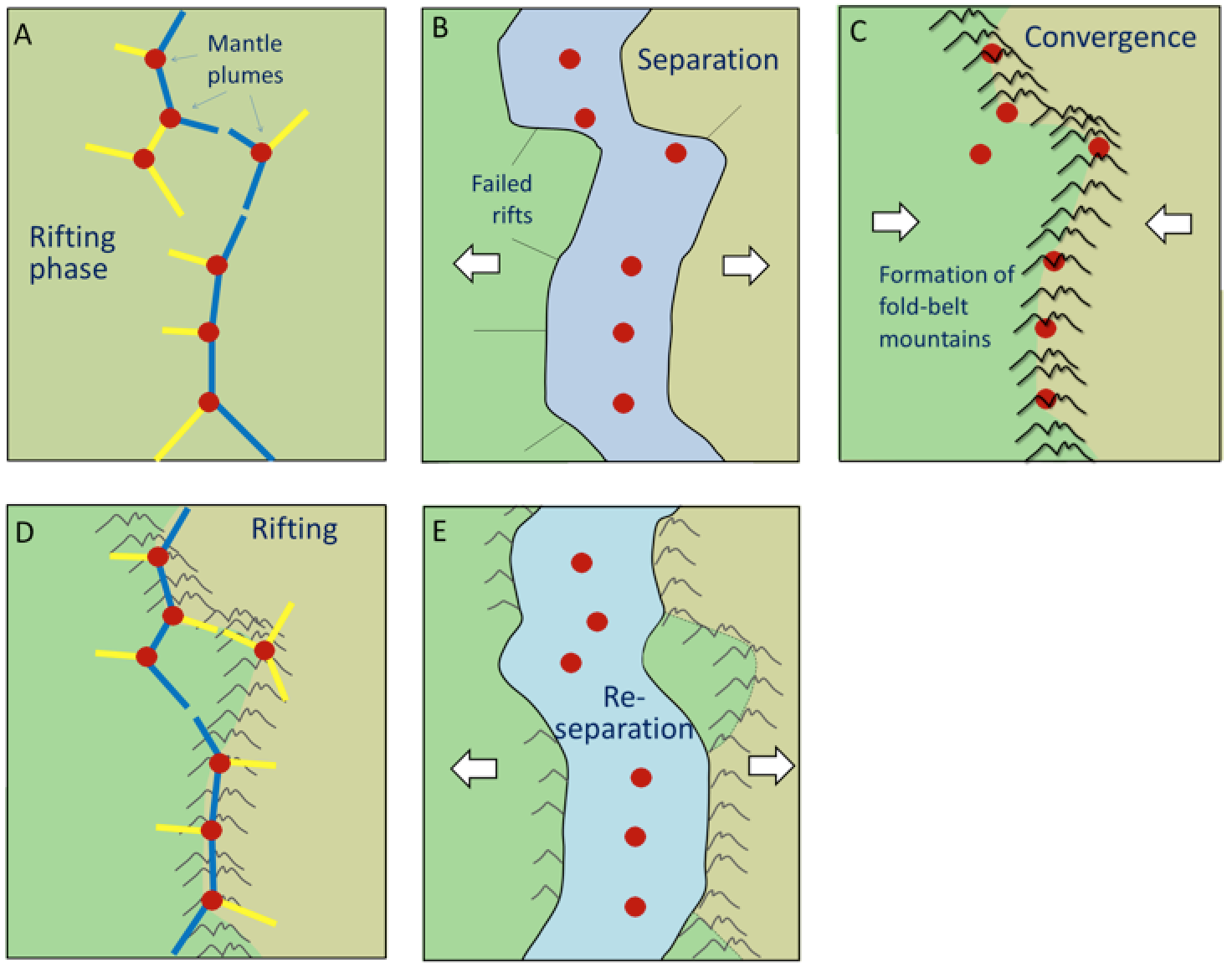
Exercise 10.5 Getting to know the plates and their boundaries
This map shows the boundaries between the major plates. Without referring to the plate map in Figure 10.4.1, or any other resources, write in the names of as many of the plates as you can. Start with the major plates, and then work on the smaller ones. Don’t worry if you can’t name them all.
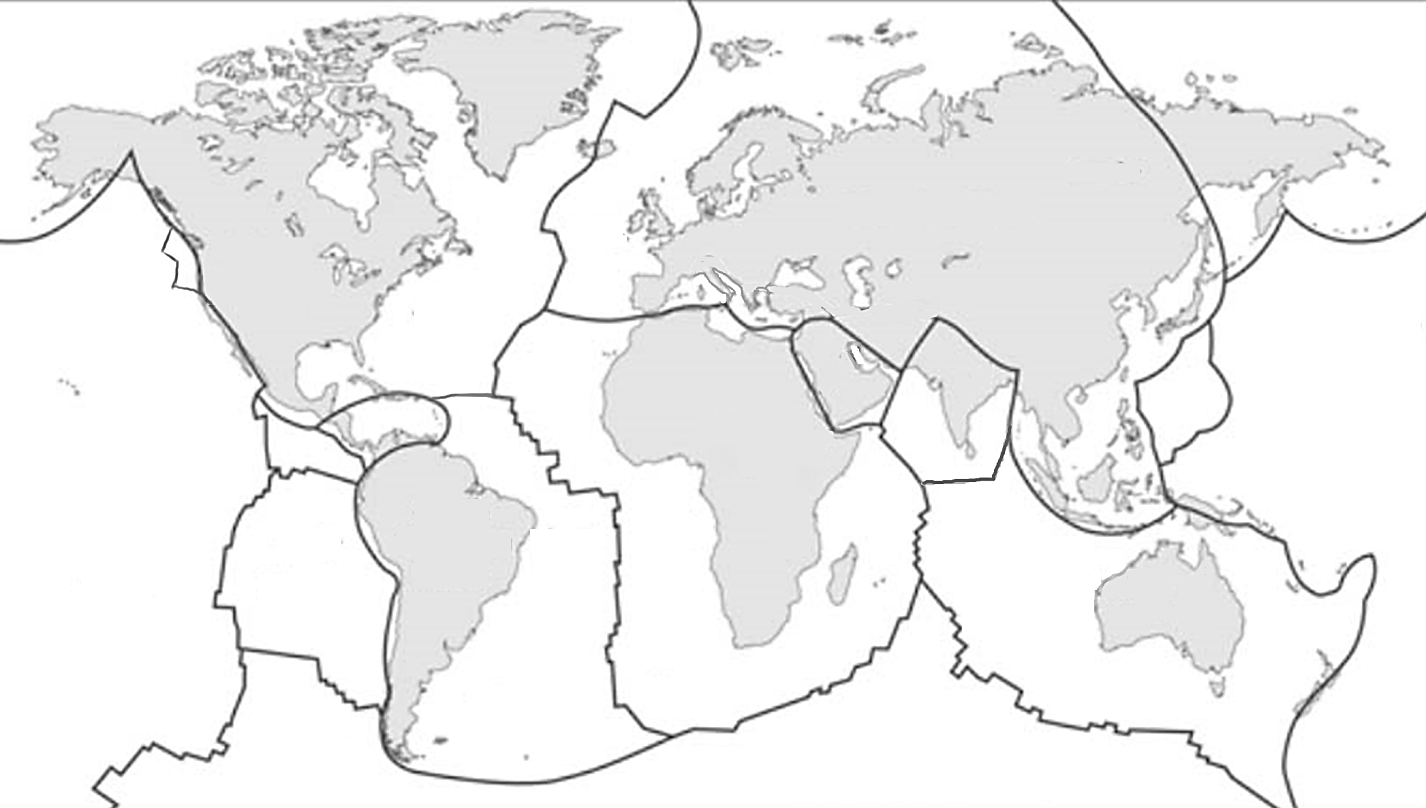
Once you’ve named most of the plates, draw arrows to show the general plate motions. Finally, using a highlighter or coloured pencil, label as many of the boundaries as you can as divergent, convergent, or transform.
See Appendix 3 for Exercise 10.5 answers .
Image descriptions
[Return to Figure 10.4.1]
Media Attributions
- Figure 10.4.1: “ Plates tect2 en ” by the USGS . Adapted by Steven Earle. Public domain.
- Figures 10.4.2, 10.4.3, 10.4.5, 10.4.6, 10.4.7, 10.4.8, 10.4.9, 10.4.10, 10.4.11, 10.4.12, 10.4.13, 10.4.14: © Steven Earle. CC BY.
- Figure 10.4.4: © Steven Earle. CC BY. Based on Keary and Vine, 1996, Global Tectonics (2ed), Blackwell Science Ltd., Oxford.
a plate boundary at which the two plates are moving towards away from each other
a plate boundary at which the two plates are moving towards each other
a boundary between two plates that are moving horizontally with respect to each other
a series of near-vertical dykes formed in the vicinity of a spreading ridge when magma from depth flows into fractures formed by extensional forces
the steeper part of a continental margin, that slopes down from a continental shelf towards the abyssal plain
the supercontinent that existed between approximately 300 and 180 Ma
a boundary between a continent and an ocean at which there is no tectonic activity (e.g., the eastern edge of North America)
Physical Geology - 2nd Edition Copyright © 2019 by Steven Earle is licensed under a Creative Commons Attribution 4.0 International License , except where otherwise noted.
Share This Book

IMAGES
VIDEO
COMMENTS
Most movement occurs along narrow zones between plates where the results of plate-tectonic forces are most evident. There are four types of plate boundaries: Divergent boundaries -- where new crust is generated as the plates pull away from each other. Convergent boundaries -- where crust is destroyed as one plate dives under another.
Figure 10.4.1 10.4. 1 A map showing 15 of the Earth's tectonic plates and the approximate rates and directions of plate motions. Rates of motions of the major plates range from less than 1 cm/y to over 10 cm/y. The Pacific Plate is the fastest, followed by the Australian and Nazca Plates.
Key points: Earth's lithosphere, or outermost shell, is broken up into large pieces called tectonic plates. These plates move slowly over the asthenosphere, a layer of softer rock below the lithosphere. On average, tectonic plates move a few centimeters per year. The place where two plates meet is called a plate boundary.
Principles of plate tectonics. A cross section of Earth's outer layers, from the crust through the lower mantle. In essence, plate-tectonic theory is elegantly simple. Earth 's surface layer, 50 to 100 km (30 to 60 miles) thick, is rigid and is composed of a set of large and small plates. Together, these plates constitute the lithosphere ...
PLATES AND PLATE MOTION (3) A plate is a rigid slab of rock that moves as a unit. As a result, the interior of plates tend to be relatively inactive tectonically. Plate interiors generally lack earthquakes, volcanoes, young mountain belts, and other evidence of geologic activity. According to plate tectonic theory, these features are caused by ...
Rates of motions of the major plates range from less than 1 cm/y to over 10 cm/y. The Pacific Plate is the fastest at over 10 cm/y in some areas, followed by the Australian and Nazca Plates. The North American Plate is one of the slowest, averaging around 1 cm/y in the south up to almost 4 cm/y in the north. Plates move as rigid bodies, so it ...
1.1 Introduction. Plate tectonics is the grand unifying theory in geology. It gets that title because many topics in geology can be explained, in some way, by the movement of tectonic plates. Tectonic plates are composed of Earth's crust and the uppermost, rigid portion of the mantle.
Plate boundaries and driving mechanisms. Graeme Eagles, in Regional Geology and Tectonics (Second Edition), 2020. Summary. Plate motion is maintained due to the detailed balance of (1) buoyancy forces generated by their thickness variations, by the distribution and size of their subducted parts and by upwelling in the mantle beneath them, with (2) resistance at their various interfaces with ...
We can credit Alfred Wegener (Figure 4.1.1) as the originator of this idea. Figure 4.1.1 Prof. Dr. Alfred Wegener, ca. 1924-1930 (Public domain, via Wikimedia Commons). Alfred Wegener (1880-1930) earned a PhD in astronomy at the University of Berlin in 1904, but he had always been interested in geophysics and meteorology and spent most of his ...
Plate tectonics is a scientific theory that explains how major landforms are created as a result of Earth's subterranean movements. The theory, which solidified in the 1960s, transformed the earth sciences by explaining many phenomena, including mountain building events, volcanoes, and earthquakes. In plate tectonics, Earth's outermost layer, or lithosphere —made up of the crust and ...
For example, take the Great Rift Valley in Africa. The African, Arabian, and Indian plates are moving. The land of Africa east of the Great Rift Valley is separating from the rest of Africa. Eventually (a few million years), a new plate will be created, being the land that has separated. ( 8 votes)
Plate tectonics (from Latin tectonicus, from Ancient Greek τεκτονικός (tektonikós) 'pertaining to building') is the scientific theory that Earth's lithosphere comprises a number of large tectonic plates, which have been slowly moving since about 3.4 billion years ago. The model builds on the concept of continental drift, an idea developed during the first decades of the 20th century.
The plates make up Earth's outer shell, called the lithosphere. (This includes the crust and uppermost part of the mantle.) Churning currents in the molten rocks below propel them along like a ...
Conclusion. Plate tectonics is the grand, unifying theory of Earth sciences, combining the concepts of continental drift and sea-floor spreading into one holistic theory that explains many of the major structural features of the Earth's surface. It explains why the oceanic lithosphere is never older than about 180 Ma and why only the continents ...
While the movement of tectonic plates is usually slow—typically just a few centimeters per year—plate tectonics are linked to several kinds of natural disasters, namely earthquakes, volcanoes, and tsunamis. On the afternoon of March 11, 2011, a large earthquake struck off the northeastern coast of Japan. This event, which would prove to be ...
• correctly illustrate the motion of three types of plate motion. (transform, divergent, and convergent) • draw arrows that correctly illustrate the motion of three types of plate motion (transform, divergent, and convergent); and • write a compare and contrast essay describing the type of surface features found at each plate boundary.
The sources of plate motion are a matter of intensive research and discussion among scientists. One of the main points is that the kinematic pattern of the movement itself should be separated clearly from the possible geodynamic mechanism that is invoked as the driving force of the observed movement, as some patterns may be explained by more ...
After reading this essay you will learn about the plate tectonics theory. Plate Tectonic theory is based on an earth model characterized by a small number of lithospheric plates, 70 to 250 km (40 to 150 mi) thick, that float on a viscous under-layer called the asthenosphere. These plates, which cover the entire surface of the earth and contain ...
We would like to show you a description here but the site won't allow us.
Plate Tectonics and Landform Processes The Aleutian Islands, Alaska The Aleutian Islands are located along the southwestern coast of Alaska, ad at the northern edge of the Pacific plate. This plate runs along the Pacific coast of North America, with its well-known faults -- the San Andreas and the Denali -- causing the strike-slip plate motion that is familiar to residents of the Pacific ...
slowly moving apart in different directions. a sudden shaking of earths surface. Some images used in this set are licensed under the Creative Commons through . Click to see the original works with their full license. Study with Quizlet and memorize flashcards containing terms like PM Key Concept #1, PM Key Concept #2, PM Key Concept #3 and more.
Figure 10.4.1 A map showing 15 of the Earth's tectonic plates and the approximate rates and directions of plate motions. [Image Description] Rates of motions of the major plates range from less than 1 cm/y to over 10 cm/y. The Pacific Plate is the fastest, followed by the Australian and Nazca Plates. The North American Plate is one of the ...
These videos are used in conjunction with the Amplify Science Plate Motion curriculum created for North Lake Middle School 7th Grade science classes.